4.1 Introduction
Fish are in continuous contact with a wide variety of nonpathogenic and pathogenic microbes present in the aquatic environment, and therefore, have developed mechanisms to guarantee their survival. The normal structure and function of the fish integument reflects the adaptation of the organism to the physical, chemical, and biological properties of the aquatic environment, and the natural history of the organism. Vertebrate skin with its outermost layer, the epidermis, covers the outer surface of a fish, including the body and fins, separates the individual from its environment, and is one of the organism’s crucial interfaces for contact and communication with its external milieu. The skin, sometimes referred to as the integumentary system, is the largest organ of the body. It has a complex structure, being composed of many different tissues. Skin is a multipurpose tissue that encases the body and serves numerous vital functions such as maintaining the body shape, protecting the fish from insults such as physical damage and microorganism invasion, and preservation of hydrodynamics. It also performs many functions that are important in maintaining physiological homeostasis such as osmotic balance. Mucous glands, which offer protection from microorganisms, are extremely numerous in fish skin, especially in cyclostomes and teleosts. Protection from abrasion and predation is another function of the fish skin, and dermal (skin) bone arose early in fish evolution in response to this need. It is thought that bone first evolved in skin and only later invaded the cartilaginous areas of the body to provide additional support and protection. Skin is well supplied with blood vessels and nerve endings that receive tactile and thermal stimuli. Some fish breathe, in part through the skin, by the exchange of oxygen and carbon dioxide between the surrounding water and numerous small blood vessels near the skin surface. Skin also serves as protection through the control of coloration. Fish can exhibit an almost limitless range of colors, which allow fish to blend closely with their surroundings, efficiently hiding the animal from predation. Skin is also a site of mucosal immunity. The fish immune system can be subdivided according to the anatomical location. The mucosal-associated lymphoid tissue (MALT) in teleost fish is subdivided into gut-associated lymphoid tissue (GALT), skin-associated lymphoid tissue (SALT), and gill-associated lymphoid tissue (GIALT). The innate immune response in fish is considered an essential arm in combating pathogens due to the limitations of the adaptive immune system (e.g., limited repertoire of immunoglobulins, slow proliferation, maturation and memory of lymphocytes, and influence of environmental factors such as temperature). The fish innate immune system can also be divided into three compartments: the mucosal/epithelial barrier (including skin, gills, and gut), the humoral components, and the involved cells (see Chapter 2). Skin and gills act as the first barrier to infection because they are the point of pathogen entry. Since most of pathogens start the process of infection in the mucosal surfaces, the mucosal immune response plays an essential role in the course of the infection, and different studies have begun to examine their cellular and molecular composition. Commensal microflora (normal microflora/indigenous microbiota) present on fish skin will not be discussed in this chapter (see Chapter 10) (Figure 4.1).
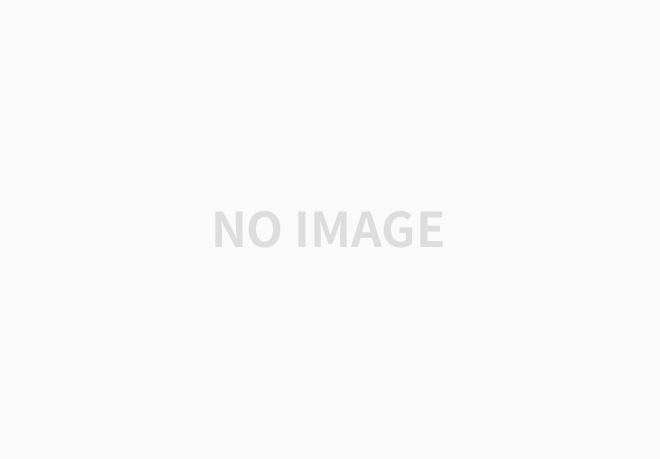
Figure 4.1 Schematic depiction of teleost fish skin highlighting the general structure, components, and cell types present. Elements that are suspected to be present in the skin, but not proven thus far are indicated as (?).
4.2 The mucous layer as a barrier against pathogens
One of the most distinctive features of fish skin is the presence of external mucus. The external mucous gel forms a layer of adherent mucus covering the living epithelial cells. This mucosal layer forms a thin barrier that separates the fish from its aquatic environment. The skin mucus is a semipermeable, physical, chemical, and biological barrier. Mucus is considered the first line of defense against infection through the epidermis (Raj et al., 2011). Skin mucus is impermeable to most bacteria and many pathogens. Mucus captures foreign particles, bacteria, and viruses, which are removed from the skin mucosa by the surrounding water. Due to this effect of mucus, pathogens are immobilized before they can adhere to or make contact with epithelial cells. Moreover, the mucous layer on the surface of the fish is not static, but dynamic both in the quantity and the quality of the substances present. Mucus is continuously secreted and replaced, which prevents colonization by potential infectious agents. Regarding the comprehensive set of innate immune defense mechanisms present at skin mucosal surfaces, the mucus is widely regarded as the most significant (Esteban, 2012). The mucous layer is secreted by epidermal cells, mainly goblet cells and club cells, which are the unicellular glands present on the epidermis. Mucous composition is very complex and it includes widely varied biologically active substances that function as humoral innate immune factors as well as playing important roles in inhibiting the entry of pathogens. The complete range of immune factors present in the skin mucus is poorly understood. The main molecules present in mucus are high molecular weight and highly glycosylated glycoproteins called mucins. These molecules play a key role in the defense of the mucosa, and confer important adhesive, viscoelastic, and rheological properties to mucosal layers. In fact, mucins act as a mechanical barrier by serving as filters for pathogens (Roussel and Delmotte, 2004). Knowledge of the immune factors present in the skin mucus and their role in the defense against external insults remains scarce. However, it is known that in teleosts the compartment of innate immune molecules is more diverse than that of mammals. The antimicrobial properties of epidermal mucus against infectious pathogens (bacteria and viruses) have been demonstrated in different fish species (Esteban, 2012). Present among these molecules are lysozymes, complement, lectins, agglutinins, calmodulin, interferons, C-reactive protein, proteolytic enzymes, antimicrobial peptides, vitellogenin, and immunoglobulins (Nigam et al., 2012). Among these substances, lysozyme is one of the most well characterized. Lysozyme is a ubiquitous bactericidal enzyme identified in a wide range of organisms, including fishes. Fish lysozyme exhibits lytic activity against both gram-positive and gram-negative bacteria; it acts as an opsonin (molecules that act as binding enhancers for the process of phagocytosis) and activates the complement system and phagocytic cells, which collectively contribute to the host defense against bacterial infection (Saurabh and Sahoo, 2008; Subramanian et al., 2007). Lysozyme activity has been shown to significantly vary depending on many environmental (such as season, photoperiod, water temperature, pH, toxins) and intrinsic (including sex, age, size, infections, stressors) properties. Furthermore, it has been demonstrated that lysozyme activity showed no significant correlation with other immune substances present in fish mucus, suggesting that lysozyme is constitutively secreted in the skin mucus of fish (Jung et al., 2012). Fish mucus also contains antimicrobial peptides (AMPs, also called host defense peptides), which are positively charged short-chain amino acid molecules involved in the host defense. Their value in innate immunity lies in their ability to function without either specificity or memory. Teleost skin is a major source of AMPs with approximately 70% of all AMPs expressed in the skin, compared to 52% and 29% expressed in the gills and the gut, respectively (Gomez et al., 2013). They are potent and broad spectrum antimicrobials with little or no toxicity to host cells, and demonstrate potential as novel therapeutic agents. Furthermore, they inhibit DNA, RNA, and protein synthesis. In the skin, AMPs prevent colonization by pathogens, and are produced and stored in granules of phagocytic cells, where they directly kill the phagocytozed pathogens (Bulet et al., 2004). They are also involved in neutralization of endotoxins, leukocyte chemotaxis, immunomodulation, angiogenesis, iron metabolism, and wound repair (Guani-Guerra et al., 2010). In fish skin, several AMPs such as hipposin, piscidin, pleurocidin, parasin, defensin, and hepcidin have been found (Najafian and Babji, 2012; Gomez et al., 2013). Due to their antibiotic properties, fish AMPs could have important applications as antimicrobial and antitumoral agents, vaccine adjuvants, and inactivated vaccines (Rajanbabu and Chen, 2011). Proteases are proteolytic enzymes that can be found in skin mucus contributing to the natural resistance of fish to infection. These enzymes are able to act directly on bacterial pathogens by cleaving their proteins, leading to death of the bacteria. Proteases also protect against pathogen invasion in an indirect manner by altering mucous consistency, thereby resulting in the increased sloughing of mucus and pathogen removal from bodily surfaces. Proteases can also activate and increase the production of other innate immune components present in fish mucus such as antibacterial peptides, complements, or immunoglobulins. Trypsin, a serine protease, cleaves peptide chains mainly at the carboxyl side of the amino acids lysine or arginine, except when either is followed by proline. Trypsin comprises more than 25% of the complement system and is reportedly one of the major mucous proteases in several fish species (Nigam et al., 2012). Other proteases, such as cathepsin B and L (cysteine proteases), cathepsin D (aspartic protease), and metalloproteases, have also been identified in fish skin mucus (Esteban, 2012). Fish mucus is also a rich source of lectins. Lectins are important immune mediators in lower vertebrates and invertebrates, and they may participate in innate or acquired immunity. These proteins are carbohydrate-binding proteins, macromolecules that are highly specific for sugar moieties. They have the ability to bind carbohydrates, which are involved in the attachment to cell walls. Lectins can block the attachment of the potential pathogen to inhibit invasion. Due to these properties lectins are considered potential antimicrobial agents in the skin mucus. Lectins are also involved in cell agglutination and/or precipitation of glycoconjugates. Lectins bind structures on the surface of pathogens, and can opsonize and enhance phagocytic activity, or result in activation of the complement pathway (Matsushita et al., 2004). Different types of lectin have been identified in the skin mucus of fish. Congerin from the conger eel (Conger myriaster) and AJL-1 from the Japanese eel (Anguilla japonica) were classified as galectins (characterized by its specific binding to b-galactoside). Congerins are produced and secreted into mucus by the club cells in the mucosal epithelium lining the skin (Kamiya et al., 1988). Other key proteins have been identified in fish mucus, and all of them have major immune functions. For example, lactoferrin is a non-heme iron-binding protein that is part of the transferrin protein family. In addition to inducing systemic immunity, lactoferrin can inhibit allergic responses and promote skin immunity (González-Chávez et al., 2009). Calpain and keratin have also been reported in different tissues of fish (Rajan et al., 2013; Salem et al., 2004). Mucosal-specific calpains present in higher vertebrates are thought to play important roles in mucosal defense, whereas keratin is a cytoskeletal protein whose primary function is to protect cells from mechanical and nonmechanical injuries. Recent reports have also shown that keratin from fish mucus possesses antibacterial activity owing to its pore-forming properties (Molle et al., 2008). Peroxidase activity has been recently demonstrated in the skin mucus of a marine teleost, and significant differences in peroxidase activity were found between skin mucus and serum samples from the same specimens, which may suggest that this enzyme has an important role in mucosal defense. Other enzymes such as alkaline phosphatase or esterase have also been identified in fish skin mucus (Guardiola et al., 2014). Immunoglobulins (Ig), the primary humoral component of the acquired immune system, can also be found in skin mucus, and play a pivotal role in the maintenance of mucosal homeostasis (Brandtzaeg, 2009). Moreover, antibodies in cutaneous mucus and the skin of teleosts play a critical role in the protective host defense against surface infections. Up to now, three Ig classes have been identified in teleosts: IgM, IgD, and IgT (also called IgZ in some species) (Salinas et al., 2011; Sunyer, 2013). IgM is the most common Ig in the plasma of teleosts and is the principal Ig involved in systemic immunity. IgM is usually found in high concentrations in fish serum; however, it has been reported to be present at very low levels (from 8 to 90 mg/mL) in the skin mucus of several fish species (Hatten et al., 2001; Zhao et al., 2008). It is thought that the IgM antibodies possess a limited antigen spectrum in fishes. Furthermore, their presence depends on their spatial distribution; Ig levels in channel catfish were found to be highest on lateral skin, lower between pectoral and anal fins, and lowest on the caudal fin and ventral skin (Zilberg and Klesius, 1997). In the earliest studies, no apparent differences between Ig present in skin mucus and serum were reported. More recently, two Igs of different molecular weights were observed in the skin mucus of sheep head. In carp, it was described that the Ig from cutaneous mucus had different protein/carbohydrate composition and antigenicity from that of serum IgM. This tetrameric cutaneous Ig may correspond to one of the two more recently identified carp IgZ (IgZ1 and IgZ2) (Ryo et al., 2010; Savan et al., 2005). A unique redox form consisting of halfmeric constituents (H1L1, ∼100 kDa) has been reported in rainbow trout skin mucus (Bromage et al., 2006). IgT, similar to mammalian IgA, is the only teleost Ig isotype with a specialized mucosal function as demonstrated in the gut of rainbow trout (Zhang et al., 2010). In addition, it has been demonstrated that IgT plays the prevailing role in skin mucosal immunity, being the most abundant Ig isotype in the skin mucosa of rainbow trout (Xu et al., 2013). It is expected that additional novel substances present in fish mucus will be described in coming years due to the emerging interest in mucosal fish defense as well as new possibilities of discovering important molecules for human medicine.
4.3 Resident cell types found in the skin
In general, the skin of adult teleosts is divided in three layers: the cuticle or mucous layer, the epidermis, and the dermis. In fish, the outermost layer of cells is alive and it retains the capacity to divide. In that regard, teleost epidermis is a stratified epithelium of variable thickness consisting entirely of live cells, of which the majority is squamous cells and the minority is mucous cells. Squamous cells are characterized by numerous desmosomes and associated cytoplasmic filaments. Teleost epidermis can be subdivided into surface, intermediate, and basal layers. The surface layer is a single-celled layer of squamous cells with only minimal quantities of keratin that develops microridges at the outer surface. These microridges contain mucus and antibacterial substances secreted to the surface from mucous goblet cells located in the intermediate stratum of the epidermis. Cells within this epidermal layer are not periodically renewed, but are replaced individually on cell death. The intermediate layer of epidermis is composed of various types of cells, including but not limited to unicellular glands (such as mucous cells and club cells), sensory cells, ionocytes, immune cells, pigment cells, and undifferentiated cells. The basal part of the epidermis is a single-cell layer (basal layer), which is attached to the basement membrane via hemidesmosomes. The basal layer tightly links the epidermis to dermis (Chang and Hwang, 2011; Esteban, 2012; Salinas et al., 2011). The dermis is mainly composed of dense connective tissue with a large amount of collagen fibers, although it typically contains relatively little of the connective tissue found in tetrapods. Instead, in most species, it is largely replaced by solid, protective bony scales. In place of true scales, cartilaginous fishes have numerous tooth-like denticles embedded in their skin. The hypodermis consists of both loosely organized collagen fibers and a rich supply of blood vessels and, as the innermost layer, is closest to the striated muscle underneath the skin (Esteban, 2012).
4.3.1 Leukocytes
In contrast to mammals, fish lack major lymphoid accumulations in mucosa-associated tissues. Nevertheless, skin-associated lymphoid tissue (SALT) contains a variety of leukocytes, including, but not limited to, lymphocytes (T and B cells), plasma cells, macrophages, and granulocytes. Leukocytes and other amoeboid cells can migrate through normal mucous secretions (Esteban, 2012).
4.3.1.1 Myeloid cells
Mast cells, also known as eosinophilic granular cells (EGCs), are tissue-resident cells found throughout the body, particularly in association with structures like blood vessels and nerves that are in proximity to surfaces that interface with the external environment. In fish, mast cells are found in a variety of tissues, including the skin, gut, gills, brain, and in the vicinity of blood vessels. Functionally, teleost EGCs show close similarity to the mast cells of mammals. Mast cells may play an important role in the inflammatory response because they express a variety of functional proteins, including antimicrobial peptides that act against a broad spectrum of pathogens. In fish, mast cells from mucosal tissues have been studied with respect to their AMP content. However, little is known about the biology or function of these innate immune cells in skin mucosa (Esteban, 2012; Gomez et al., 2013). In mammals, dendritic cells (DCs) act as messengers between the innate and adaptive immune systems. In different tissues, these cells act as sentinels of the immune system. When a pathogen is located and engulfed, antigens are transported to the secondary lymphoid tissues and expressed, which stimulates the production of specific T lymphocytes. Although DCs are of fundamental importance in the mammalian immune response, their presence and function in nonmammalian vertebrates has been poorly studied. Recently, dendritic-like cells have been characterized in zebrafish and rainbow trout, and it has been postulated that zebrafish skin contains DCs that are equivalent to mammalian Langerhans cells (Bassity and Clark, 2012; Lugo-Villarino et al., 2010). Macrophages and various types of granulocytes, such as neutrophils, are also present in the skin mucosal lymphoid tissue, playing a key role as sentinel cells of the innate immune response. They are phagocytic myeloid cells; therefore, they are involved in different processes such as homeostatic mechanisms, wound healing, and the detection, elimination, and clearance of foreign entities including tumors, virus-infected cells, and invading pathogens. Moreover, macrophages and neutrophils produce hundreds of bioactive molecules that play relevant roles in the processes of pathogen recognition and destruction, cellular communication and activation, initiation of an adaptive immune response, and resolution of the inflammatory response and tissue repair (Katzenback et al., 2012).
4.3.1.2 Lymphoid cells
B and T cells are adaptive immune elements detectable in the skin of both cartilaginous and teleost fish. Among other functions, the main role of B cells in adaptive immunity appears to be the recognition of antigens in their native form and the production of Igs against those antigens. In carp skin, B cells have been detected only in the epithelium. In spotted wolffish, by using in situ hybridization, IgM+ cells were found both in the epithelium near the basal membrane and further out in the epidermis (Grontvedt and Espelid, 2003). As B cells are able to produce three types of Igs (IgM, IgD, and IgT/IgZ), there are different B cell subsets based on the Igs that they express. Some teleosts like the channel catfish possess three B cell subsets IgM+/IgD+, IgM+/IgD-, IgM-/IgD+ (Edholm et al., 2011), whereas two populations (IgD+/IgM+/IgT- and IgD-/ IgM-/IgT+ B cells) have been characterized in rainbow trout (Zhang et al., 2010). Although both IgT+ and IgM+ B cells are present at low percentages in the skin of trout, IgT+ B cells represented the main skin B cell subset in this tissue (Xu et al., 2013).
T cells play an essential role in cell-mediated immunity, and as they interact with the bacteria present on mucosal surfaces, it seems they are very important in creating tolerance or immunity against the commensal microbiota. There are different T cell subsets in mammals (cytotoxic, Th1, Th2, Treg, and Th17) and some of them have also been described in teleost fish. However, teleost skin T cells have not been studied in detail so far (Gomez et al., 2013).
4.3.2 Epithelial cells
The basic cellular element of the fish epidermis is the epithelial cell. Epithelial cells are active orchestrators of homeostasis, commensal colonization, and innate and adaptive immune response through the expression of PRRs, including lectins, NOD-like receptors (NLRs), and toll-like receptors (TLRs). Moreover, they are capable of interacting directly with pathogens and commensal bacteria (Gomez et al., 2013). Contrary to mammals, the epithelial cells in teleost fishes are alive and metabolically active, so they retain the capacity for mitotic division. Cells in any layer of the epidermis can potentially divide, although mitotic activity is most common in the lower layers. In this way, dead cells are regularly sloughed from the epidermal surface and replaced by living cells beneath. The exterior surface of superficial epithelial cells of teleosts is characterized by microridges, which aid in retaining mucous secretions on the skin surface. The epithelial cell is the key element in the unique wound repair mechanism of fish skin. Shortly after damage occurs, epithelial cells migrate from the edges of the wound toward the wound cavity in compact groups. These cells can rapidly cover the wound, providing a protective barrier against infection by opportunistic pathogens during the early stages of wound repair (Elliott, 2011).
4.4 Skin immune responses in diseased fish
Fish are in an intimate contact with their environment and as compared to terrestrial animals, fish “swim” in a sea of pathogens. Thus, any rupture in the normal barrier function of the skin could allow colonization of the skin by infectious agents or invasion by opportunistic microorganisms (microorganisms that normally colonize the skin but are typically of low pathogenicity) (Law, 2001). For this reason, cutaneous disease is relatively more common in fish than in terrestrial vertebrates. Fish skin possesses highly developed antimicrobial features, many of which have been preserved throughout evolution, and immune defense strategies employed by piscine skin are still operative in human skin. The process of pathogen entry through the skin is being studied by using noninvasive whole-body imaging techniques on living animals. Bioluminescence imaging, typically using luciferase reporter systems/constructs, is now widely used in small-animal models such as rodents and fish. This technique offers the advantage of using the same animal for multiple data collections over the course of the entire experiment. Moreover, luciferin, the substrate of luciferase, has been shown to cross cell membranes allowing this reporter protein to be imaged in different tissues of interest (Costes et al., 2009). Some observed differences in disease susceptibility between fish species and/or strains have been linked to the differing ability of the fish to prevent pathogen attachment and entry at mucosal epithelial sites. Cutaneous lesions are generally nonspecific and may be indicative of disease that is restricted to the integument or a manifestation of systemic disease. Fish skin is able to react uniquely to different attacks. Changes in the skin are “a priori,” the most readily observed clinical features of fish, including traumatic lesions, changes in coloration, and/or changes in one or more parts of the skin (including both humoral factors and different cell types). The perfect combination of mucous composition and the kinetic processes of the epidermis must prevent colonization of the skin surface and, for this reason, prevent infection. In the next subsections, the main changes in the skin mucus as well as those observed in skin epidermis and dermis in response to infection will be described.
4.4.1 Changes in the skin mucus in response to infection
As described above, skin mucus has powerful mechanisms that can immobilize or even kill pathogens before they contact epithelial surfaces. Changes in the amount of mucus (an increase or decrease) are common but the most interesting changes are those affecting the chemical composition of the mucus. These changes, due to the large number of substances that can be involved (many still uncharacterized), are responsible for the varied responses that orchestrate mucosal skin immunity. Furthermore, all of these changes can take place without the pathogen in question making physical contact with the epithelial cells underlying the layer of mucus. The mechanisms of pathogenicity induced by certain bacteria are still uncertain and frequently those mechanisms are correlated to the ability of bacteria to adhere to mucus (Baffone et al., 2006; Zorilla et al., 2003). Vibrio alginolyticus strains show a specific binding capability to gilthead sea bass and gilthead seabream mucus (Snoussi et al., 2008). A strong ability to adhere to the fish skin mucus of different species has been reported for different bacteria pathogenic for fish (e.g., Flavobacterium columnare; Photobacterium damselae, subsp. damselae; V. vulnificus; V. alginolyticus; V. anguillarum; Aeromonas hydrophila; P. damselae, subsp. piscicida; and Flexibacter maritimus) (Fouz et al., 2000). However, other studies have demonstrated that the skin mucus of some fish species is able to inhibit the adhesion of certain pathogenic bacteria. For example, seabream skin mucus inhibits the adhesion of Pasteurella piscicida, F. maritimus, V. anguillarum, and V. damsela. Some pathogens have developed strategies to circumvent the mucosal barrier to reach epithelial cells. Specifically, the mucous layer can be amended by the pathogen, allowing the pathogen to move through the mucus and adhere to the epithelial cells (Cone, 2009). In the skin of channel catfish and blue catfish, A. hydrophila infection rapidly altered the gene expression of a number of potentially critical lectins, chemokines, interleukins, and other mucosal factors in a coordinated manner predicted to enhance its ability to adhere to and invade catfish hosts (Li et al., 2013a; Li et al., 2013b).
It has also been demonstrated that some bacteria harvested from fish skin are able to degrade the skin mucus of the same fish species (Snoussi et al., 2008). For this reason, their presence in skin mucus can make the fish more vulnerable to colonization by other pathogenic or even opportunistic microorganisms. Furthermore, maintaining homeostasis of the physicochemical factors of mucus is important to avoid the potential invasion and/or adhesion of pathogens to mucosal surfaces. For example, mucous transport requires well-regulated viscoelasticity, which is controlled by hydration. By making the mucus slightly more hydrated, the fluid could have a markedly lower viscoelasticity and be readily penetrable by motile bacteria (Cone, 2009). In fact, in challenge experiments with bacteria, removal of mucus/epidermal cells increased the cumulative mortality in salmonids compared to undamaged fish (Madetoja et al., 2002). The thickness of the mucous blanket is determined by the balance between the rate of secretion and the rate of degradation and shedding. Toxic and irritating substances can greatly stimulate mucous secretion, increasing the thickness of the mucous blanket. Similarly, many infectious agents can modulate the thickness, or alter mucous layer consistency (apparent when studying fish under polarized light). This effect is particularly evident in protozoal and other parasitic infections. It is known that a mucous blanket is secreted from goblet cells in the epithelium immediately after the first contact with pathogens. More recently, it was demonstrated (by using histological, histochemical, and biochemical techniques) that carp skin mucosa responds rapidly to high bacterial loads, even if the bacteria involved are considered to be nonpathogenic, with an increased secretion of mucin molecules, especially low-glycosylated variants (Van der Marel et al., 2010). Different studies seem to corroborate that mucin-type molecules excreted by intestinal goblet cells are highly species-specific (e.g., sulphated mucins in shi drum (Umbrina cirrosa), or neutral mucins in common dentex (Dentex dentex)). To date, no similar studies have been reported in fish skin mucus. Skin mucus also acts as a medium in which antibacterial effectors may act. The mucosal skin defense system secretes different molecules or components into the mucous layer, although there is still a limited knowledge about these defense mechanisms. There are both intrinsic and constitutive antimicrobial components of the mucus (Ellis, 2001). Furthermore, mucus can also be the vehicle for other innate immune humoral factors secreted in the epidermis or in the dermis. In summary, fish skin mucus features an arsenal of a variety of naturally active substances as well as numerous defense molecules of both the innate and acquired immune system (Palaksha et al., 2008; Subramanian et al., 2007, 2008). These antimicrobial properties of epidermal mucus against infectious pathogens (mainly bacteria and viruses) have been demonstrated in different fish species (Kuppulakshmi et al., 2008) and increased expression of one or more of the above-mentioned antimicrobial components in fish epidermal mucus has been observed following microbial stress (Patrzykat et al., 2001), thus supporting the function of epidermal mucus in defending fish from infectious pathogens. Among the humoral factors present in fish mucus in the present chapter, we will describe the variations of some enzymes, proteases, antimicrobial peptides, and other molecules more recently studied. Regarding enzymes, the levels of some of them increased in skin mucus as a consequence of injury and/or infection. For example, increased activities of phosphatases (considered to play a protective role) were demonstrated in epidermal cells after and during skin injury and regeneration related to cutaneous wound healing, during stress or parasitic infections. Furthermore, alkaline phosphatase has been demonstrated as a potential stress indicator in skin mucus of Atlantic salmon (Ross et al., 2000). It seems that no significant relationship was observed between the phosphatase activity and other mucosal parameters (Jung et al., 2012). Secretion of proteases into skin has been described as a consequence of the pathogen recognition. Proteases may act directly on a pathogen (e.g., they can kill bacteria by proteolysis) or may modify mucous consistency or increase the sloughing of the mucus, effectively preventing pathogen invasion and facilitating the removal of pathogens from the bodily surfaces. Proteases also activate and enhance the production of other known innate immune components present in fish mucus (such as complement, antibacterial peptides, or Ig). Antimicrobial peptides are gradually more recognized as a significant component of the host’s defense against infection and they are considered a very important part of the mucus and skin barrier function. AMPs are produced (constitutively or induced upon infection in fish epidermal mucus) to defend against invading pathogens (Sarmas¸ik, 2002). Lectins interact with pathogenic surface structures (such as those present on bacteria, viruses, or parasites) that result in increased phagocytosis (through opsonisation) or complement activation. Lectins recognize different pathogenic bacteria. For example, congerins recognize marine bacteria such as V. anguillarum. Similarly, pufflectin binds to the parasitic trematode (Heterobothrium okamotoi). Pufflectin (a mannosespecific lectin isolated from the skin mucus of pufferfish) shows no sequence similarity with any known animal lectins, but unexpectedly shares sequence homology with mannose-binding lectins found in monocotyledonous plants. Recently, a rhamnosebinding lectin expressed in gill/skin was found to play a role in the pathogenesis of columnaris disease in channel catfish Ictalurus punctatus (Beck et al., 2012). Intelectin protein is expressed in skin and gill club cells of catfish (Silurus asotus). Although the intelectin showed agglutination activity against the pathogenic bacterium A. salmonicida, its expression was not induced by in vivo bacterial stimulation. Taken together all the available results on lectins present in fish mucus, it seems reasonable to think that mucous lectins in fish have wide molecular diversity (Suzuki et al., 2003). Furthermore, there is evidence that suggest that lectins actively participate in the self-defense system by acting on intra- and extra-body surfaces. The antimicrobial effect of histones is now well known, but only recently are they being recognized as endogenous antibiotics and, for this property, being linked to the innate immune system of fish (Fernandes et al., 2002, 2003). Histone H2B is present in the skin mucus of Atlantic cod and inhibits important bacterial and fungal pathogens of fish (e.g., A. hydrophila and Saprolegnia spp.). Curiously, histone fragments can also show antimicrobial properties. For example, levels of N-terminal segments of catfish H2A were increased in the epidermal mucus upon stimulation (Patrzykat et al., 2001) and reduced in the absence of disease (Robinette and Noga, 2001). The available literature describing the skin mucosal immune response after viral infections is particularly scarce, but it has been demonstrated that AMPs are involved in such responses. It is assumed that viruses bearing capsids can disseminate freely through mucus to effectively enter a fish and cause disease. Thus, capsid viruses appear to have a unique mechanism to enter mucus because they are small enough, neutral in net surface charge, and are coated densely with charged groups that prevent hydrophobic binding to mucins. In addition, they have evolved effective methods for selectively adhering to and entering their target cells (Cone, 2009). However, this is not true for all the viruses. For example, it has been demonstrated that skin mucus of Cyprinus carpio inhibits cyprinid herpes virus 3 binding to epidermal cells (Raj et al., 2011). Three different b-defensins from rainbow trout increase in expression during bacterial and simulated viral challenges (Casadei et al., 2009). It is important to underline that AMPs have a double function in antiviral defense, acting not only directly on the virion, but also on the host cell. However, only a few successful attempts to assess the antiviral activities of fish AMPs have been reported. Fish depend more greatly on their innate immune defenses than mammals, and for this reason, they are seen as a likely rich source of antiviral compounds for fighting not only viral infections in fish, but potentially mammalian viruses (Falco et al., 2009). Future research will add to the understanding of the relationships between viruses and fish skin mucosa, as well as the mucosal immune responses elicited by them. The literature available on the responses of fish skin against parasites is more abundant than those available for bacteria and viruses. The skin is a very frequent place of parasite invasion despite the barrier functions associated with mucosal epithelia of fish. To minimize the effect of parasitic infection, both innate and adaptive defense mechanisms have to be involved (Jones, 2001). Here, special attention will be paid to ectoparasites, those inhabiting within or on the skin. Fish can also respond to a parasitic infection by altering the quantity or quality of the mucous secretion and this mucus plays a very important role in limiting the parasite load. Two ectoparasitic taxa have been widely studied and have contributed to the information about host resistance in fish: the gyrodactylid monogeneans and the caligid copepods. For example, infection by the parasite Trichodina or the metazoan Gyrodactylus can provoke excessive mucous production. Hypersecretion of mucus may be associated with a localized epithelial cell hyperplasia or inflammation (both reactions will be described in the next section of this chapter). Pathogenesis in gyrodactyliasis may be related to skin mucification or to a local reduction of mucous cells, and it is considered that a high mucous turnover may be involved in protecting fish against invasion in a similar way to that described for bacteria. The ciliate parasite Ichthyophthirius multifiliis (which is a common obligate and a highly motile ectoparasite) is one of the most important protozoan pathogens of freshwater fish. Parasitized fish usually die following infection, but animals surviving sublethal parasite exposure become resistant to subsequent challenge. This resistance correlates with the presence of humoral antibodies in the sera and also in the cutaneous mucus of immune fish. In fact, fish vaccinated against I. multifiliis by intraperitoneal injection of purified immobilization antigens (i-antigens; the surface proteins targeted by immobilizing antibodies) in Freund’s complete adjuvant built up active protective immunity and produced antibodies against i-antigens (Clark et al., 2001) in both the blood and the cutaneous mucus. It has been observed that parasites rapidly leave the skin of I. multifiliis-immune fish and the explanation could be an antibody-mediated mechanisms of cutaneous immunity (Dickerson and Clark, 1998). Although there is experimental evidence supporting the existence of a separate mucosal immune system in fish, it is not known exactly how mucosal antibodies reach the surface epithelia of the skin. Findings suggest that parasite-specific antibodies in the cutaneous mucus of channel catfish do not arise by passive transfer or exudation from the blood. Maki and Dickerson (2003) found that mucosal antibodies were produced following either I. multifiliis infection or the injection of purified antigen and that in both cases their occurrence did not exactly coincide with serum antibody production. In addition, when skin explants from channel catfish immunized against I. multifiliis are cultured in vitro, they release I. multifiliis-specific antibodies, implying that antibodies are actively produced by cells in skin rather than diffusing from serum (Xu et al., 2002). However, the appearance of i-antigen-specific antibodies in both skin and serum demonstrate that cutaneous mucosal and systemic immunity are integrated (Zhao et al., 2008). Despite the limited amount of related research to date, it seems that a certain parallelism exists between the response observed in the mucus of a possible bacterial and parasitic infection. Further work will help verify this assumption.
4.4.2 Changes in skin epidermis in response to infection
Most animals have the ability to repair an epidermal lesion (e.g., after an infection or a physical injury). In fish, the keratocyte participation in the epithelialization of the wound gap involves changes in the surface architecture of the epithelial cells. In fact, the epidermal healing response is very rapid in fish. As a quick response to injury, copious mucous secretion and accumulation is observed on the surface of the adjacent epithelial cells, which is also associated with the protective function of mucus against pathogenic microorganisms (Rai et al., 2012). It includes a migration of malpigian cells from the periphery of the wound over the wound surface and this makes possible the repair of the lesion. Besides this, keratocytes can cover fish skin wounds with a new protective layer of cells within hours after wounding by rapid migration from the surrounding wound margins. This capacity of fast epidermal healing of fish is crucial for avoiding subsequent infections, but even more remarkable is the property of regeneration (an exceptional and remarkable cellular event in vertebrates). Teleosts can fully regenerate largely severed appendages (such as fins) with different tissues, as it has been described in several aquatic urodelan amphibians. Fin regeneration is a rapid process in which the wound is first healed by the rapid migration and reorganization of the epithelial cells of the base to cover the surface of the cut, leading to the formation of the wound epidermis (Akimenko et al., 2003). Regarding the changes that can be observed in skin epidermis in response to infection, it is important to mention that the epidermis of fish is avascular, a characteristic that limits its range of potential responses. Frequently, changes in the epidermis reflect other pathological alterations of other tissues or organs as well as changes in the dermis (which is vascular). Pathological changes in fish epidermis include epidermal degeneration, epidermal erosion, epidermal ulceration, and leukocyte infiltration.
Epidermal degeneration is defined as swollen epidermal cells (intracellular edema) with pyknotic (condensed) nuclei. Epidermal erosion is identified by the sloughing of the epidermal layers, but with an intact basement membrane. Epidermal ulceration involves a complete loss of all epidermal layers including the basement membrane. Lastly, leukocyte infiltration is the presence of a greater number of leucocytes in the epidermal and dermal layers. At the cellular level, there are two important phenomena that determine the outcome of epithelial cell injury (similar as that which occurs in other cell types): (1) cell membrane damage (with associated fluid and ionic imbalances) and (2) the inability of mitochondria (the source of power of the cell) to restart ATP synthesis. As a tissue, fish skin, similar to most other tissues of the body, responds to injuries and severe tissue damage with an inflammatory response. Inflammation, together with hemorrhages, ulcers, and changes in coloration are the most frequent changes in the skin as a consequence of a bacterial infection. Inflammation is a protective mechanism that provides fluids to the site of injury to help preserve homeostasis in the damaged cells and tissues. The most common causes of such damage are physical and chemical traumas, microbes and their toxins, death of cells, and immune reactions. The results of acute inflammation can lead to the complete resolution of the problem, or the development of exudative or necrotic lesions and progression to chronic inflammation. When inflammation is countered rapidly, the skin will return to a normal appearance after repair by local proliferation. The earliest sign of an inflammatory response in the teleost epidermis is spongiosis (in other words, intercellular edema, separation of the cells by presence of extracellular liquid). Exudates are the results of continuous production of inflammatory response and may be of different nature: catarrhal (typical from mucous surfaces as skin), supurative (if predominantly neutrophil cells), fibrinous (if there is high leakage of fibrinogen from capillaries), or serous (abundant watery exudate in peritoneal or pericardial cavities). Necrosis occurs if cellular damage is not immediately lethal. During necrosis, intracellular fluid is accumulated and cells swell. These changes may be reversible but, if they progress, the cells may become vacuolated, and nucleic acid is damaged resulting in cell death. Epithelial cell death can also be caused by toxins produced by gram-negative bacteria (e.g., Aeromonas spp., Pseudomonas spp., V. anguillarum). Furthermore, different cell types present in fish epidermis can internalize particular matter such as bacteria and other particles. Phagocytosis is a very effective cellular innate immune activity serving to defend against microorganisms or parasites. Some bacteria (e.g., F. columnare) cause lesions in the skin, at the beginning of the infection as small places where they grow and those places appear surrounded by a hyperemic tissue giving rise to hemorrhagic ulcers and necrotic tissue. Many bacteria may also cause hemorrhages in the skin (e.g., A. hydrophila), which, when the infection progresses, may ulcerate to form necrotic lesions. Also frequent are the appearance of petechiae, which are small (1–2 mm) red or purple spots on the body, caused by a minor hemorrhages. Other bacteria cause skin ulcers featuring a discontinuity of the skin or a break in the skin, accompanied by the disintegration of tissue. Ulcers can result in a complete loss of the epidermis, portions of the dermis, and even subcutaneous fat. Skin ulcers are often visible as an inflamed tissue with an area of reddened skin. Ulcerative lesions are likely to be initiated by a series of factors that lead to a breach of the normal barrier function of the skin (Law, 2001). Skin ulcers can have many different etiologies, including infectious agents, toxins, physical causes, immunologic causes, and nutritional and metabolic perturbations. For example, the bacteria Moritella viscosa is considered to be the agent causing winter ulcer diseases characterized by extensive and chronic ulceration of the skin and septicemia (Ingerslev et al., 2010). Recently, it has been demonstrated that M. viscosa (but not A. wodanis) affected or inhibited the epidermal regeneration abilities of keratocytes (Karlsen et al., 2012). Many infectious agents can cause changes in skin pigmentation. The most frequent is skin darkening (e.g., the bacteria Flavobacterium psychrophilum, P. damselae, Pseudomonas anguilliseptica, V. anguillarum, V. salmonicida, Aeromonas salmonicida, Renibacterium salmoninarum, Mycobacterium marinum), although some other agents cause depigmentation (e.g., Edwardsiella tarda, Nocardia kampachi). Regarding the most frequent changes in the skin as a consequence of a viral infection are petechial hemorrhages and skin hypertrophy and hyperplasia. Different viruses including redfin perch iridovirus, sheatfish iridovirus, and black catfish herpes virus give rise to the petechial hemorrhages, sometimes with preference to a specific body location (e.g., in the base of the fins, operculum). One of the most studied and well-known skin responses are the changes provoked by lymphocystis disease virus. This disease is characterized by the appearance of light-colored nodular lesions on the skin. Microscopically, it was demonstrated that each nodule comprises an individual virus-infected cell, which is hypertrophied. The infected cells cease dividing, although continue growing; the cells enlarge and show a hyaline capsule before starting a degeneration phase. In this phase macrophages and other phagocytic cells surround the infected cells. Release of virus occurs when the infected cells break down and this could result in a new infection of adjacent cells. Immunohistochemistry and in situ hybridization techniques have been used for the detection of lymphocystis disease virus in different tissues. By using a polyclonal antibody raised against a major capsid protein, the virus was detected in skin dermis and caudal fin (Cano et al., 2009). White sturgeon iridovirus disease also results in the formation of numerous hypertrophied cells in the epithelium and epidermis, and infected cells are seen by light microscopy separating from the dermis. Turbot herpes virus causes giant cells in the fish malpighian epidermis cells. Furthermore, pike epidermal proliferation herpes virus can cause lesions composed of large hypertrophied cells in the fish epidermis. Hyperplasia is very common in the fish epidermis and all the cell types may proliferate. Usually, hyperplasia is accompanied by spongiosis. Hyperplasia of epithelial cells minimizes the chances of epidermal disruption. There are many causes such as chemical pollutants, hormonal stimuli, bacteria (e.g., Flexibacter sp.), virus (e.g., cod and dab adenoviruses; European catfish herpes virus provokes a thickened epidermis without mucous cells; carp pox disease provokes multiple, focal lesions of benign, noninvasive and nonnecrotizing epidermal hyperplasia; Japanese flounder herpes virus; Pacific cod herpes virus), and even, parasites. For example, Costia necator is an ectoparasite, which causes costiasis characterized by hyperplasia of the epithelial cells, exhaustion of goblet cells in the area of the parasite attachment, spongiosis, and sloughing of the epidermis. Sometimes, epidermal hyperplasia results in secondary infection with other bacteria or the abnormal tissue may be infiltrated with numerous foci of melanin. Other factors such as netting damage or skin abrasion by parasites can assist virus transmission. Most fish alloherpesviruses cause primary pathology to epithelial cells, with cells showing signs of virus replication. Characteristic histological changes in diseased fish with alloherpesvirus infections include epidermal cell necrosis (cell death), syncytia formation (multinucleated epithelial cells), epidermal cell hypertrophy, epidermal hyperplasia, and papillomas (benign tumor cells). Epithelial cells that are infected often display enlarged nuclei with marginated chromatin (Hanson et al., 2011). Regarding parasites, I. multifiliis and Gyrodactylus salaris have been used to study the ontogeny of the mucosal immune response. The infection is initiated by invasion of the skin by free swimming, ∼40 mm theronts that grow within the epithelium, causing extensive damage to the skin. Besides variations in the physicochemical characteristics of skin mucus, the presence of bioactive molecules (e.g., complement, lysozyme, C-reactive protein, lectins) and epidermal migration of inflammatory cells and their secretions may affect the establishment and propagation of parasites (Dickerson and Clark, 1998; Kania et al., 2010). There are fish parasitic protozoans that use the fish integument as their environment. While many of them are considered as ectocommensals (may simply use the skin as an anchoring substrate), they can sometimes become very numerous and may interfere with normal skin functions. Flagellated protozoans are usually ectoparasites; they attach to epithelial cells and penetrate them. For example, trophozoites from I. multifiliis and Cryptocaryon irritans destroy epithelial cells in freshwater and seawater fish, respectively. Some ectoparasites (e.g., monogenean and crustacean) modulate mucous production during attachment by reducing the density of mucous cells in the skin of the host. Pathogenesis in gyrodactyliasis is related to skin mucification or to local reduction of mucous cells. Other parasites cause deposition of melanin pigment around the encysted parasite in the host’s skin. Tumors are among the most frequently reported abnormalities involving the skin. Tumors of the epithelial tissues (including the skin) are characterized by the ability of the cells to grow in clusters or sheets of cells. Furthermore, they are also characterized by their ability to provoke the proliferation of capillaries and a stroma of connective tissue, known as a desmoplastic response. Benign tumors of epithelial cells, such as papillomas, vary in size and shape and project above the skin surface. Papillomas can be single or multiple, soft or firm, and exhibit different colors (depending on whether melanocytes are involved). Papillomas typically consist of a stratified squamous epithelium with a supporting stroma. Immunological findings tend to support histologic observations showing that lesions are ultimately sloughed as a result of a cell-mediated immune response. Papilloma cells were found to be similar to normal epidermis in having interdigitating cell membranes with desmosomes (when observed by transmission electron microscopy). Papillomas are rarely lethal, although major problems correlated to the disfigurement caused in specimens and subsequent economic loss makes the study of these tumors of interest. Clinical signs of papillomas can vary from small raised nodules to large, gray-to-black epithelial growths and can appear on any location of the body. Most epithelial papillomas are a result of viral infection (e.g., smelt papilloma herpesvirus, Atlantic salmon papilloma, Esocid lymphosarcoma, pike epidermal proliferation, or white sucker papilloma) and only in scarce cases have been demonstrated to be oncogenic. Some tumors affect only the epidermis and consist of a hyperplastic epithelium, while others affect the dermis. For example, walleye dermal sarcoma is a retrovirus causing skin lesions of nodular appearance. Although the initial stage occurs in the epidermis, in the later stages, the tumor cells affect the dermis. Piscine retroviruses comprise both exogenous and endogenous viruses; most of them are associated with proliferative diseases. Because several of these proliferative diseases have a seasonal tendency, they offer an exceptional model for studying tumor development and regression. Numerous proliferative diseases in fish have been associated with one or more retroviruses. Typically, these occur as seasonal epizootics affecting farmed and wild fish and most lesions resolve spontaneously. Natural resolution and lifelong resistance to reinfection are two features of some piscine retrovirus-induced tumors that have motivated research interest in this field. The epidemiological and morphological features of proliferative diseases in fish that have been associated with retroviruses have been recently revised (Coffee et al., 2013). Other changes visible in skin epidermis as a consequence of an infection are the presence of immune factors and immune cells involved in adaptive immunity. Regarding the adaptive immune response in fish skin, it must be taken into account some peculiarities of their immune system. The lymphatic tissue of teleosts is concentrated around the kidneys. They have neither lymph nodes nor bone marrow. However, in the higher bony fish, an adaptive, predominantly humoral immune response has been found. Skin-associated lymphatic follicles are still lacking, but now ATPase-positive dendritic cells and IgM-positive lymphocytes are seen in the epidermis (Wölfle et al., 2009). Furthermore, although the number of lymphocytes present in the epidermis is usually low, it can be highly variable depending on the severity of the immune reaction. It has also been demonstrated that allogeneic skin grafts are rejected relatively rapidly following a circadian rhythm (Flajnik and du Pasquier, 2004). Based on several experimental approaches (e.g., intraperitoneal administration of serum antibodies), it has been postulated that IgM molecules are poorly transported to the mucosal secretions. For this reason, it has been proposed that the presence of IgM in skin mucus of fish is a result of some mechanism mediating its secretion into the external fluids, and that cells localized near the skin epithelium are responsible for the production of the cutaneous antibodies. Secreted Ig is locally produced in the skin.
4.4.3 Changes in skin dermis and hypodermis in response to infection
The skin dermis and hypodermis will be considered simultaneously in terms of disease because they usually respond very similarly. In fish, cutaneous lesions are generally nonspecific and may be indicative of disease that is just restricted to the integument or a manifestation of systemic disease (Groff, 2001). In fact, there are different pathogenic microorganisms that are able to produce severe alterations to fish (in occasions, 100% mortality after infection) only by affecting the mucosal surface. For example, infection caused by the bacterium F. columnare (columnaris disease agent) causes a chronic, ulcerative, necrotic infection of the body surface. The bacteria are readily detected in skin specimens from infected fish; however, they are infrequently detected in other internal organs (e.g., liver, kidney, or spleen) (Tripathi et al., 2005). However, other bacterial infections (e.g., E. tarda) originate in the epidermis and but can progress to cause ulcers affecting the dermis, even the myonecrosis of the overlying areas characterized by cutaneous lesions that extend down into the musculature and to vital organs like the kidney or the liver. After ulceration of the skin, lesions are often hemorrhagic with fibers of collagen surrounding the infected area of the dermis. Chronic inflammatory lesions of the dermis may cause melanophore damage, lymphocytic infiltration, as well as macrophages in the epidermis that phagocytose melanosomes from damaged cells. Other bacteria can cause hemorrhagic ulcers affecting the epidermis, the dermis and the hypodermis (e.g., Pseudomonas anguilliseptica). Furthermore, bacteria like Vibrio viscosus originate subdermal focal lesions, which initially are hemorrhagic and progress to more necrotic lesions of the dermis and even of the overlying tissues. Another change observable in the dermis as a consequence of an infection is the appearance of furuncles; a good example are those caused by A. salmonicida. A furuncle results from localization of bacteria in the dermis (although sometimes in the epidermis) where the bacteria cause hyperemia with fibrinous edema and then infiltration of macrophages and granulocytes. The center of the lesions correspond to an area of liquefactive necrosis with characteristic strands of fibrin. Regarding parasitic infections, hemorrhagic and necrotic dermal lesions are often detected. In Uronema infections, appearance of brown patches on the skin coincides with the appearance of a large number of pathogens in skin. Other major clinical/ pathological manifestations include severe necrotic lesions in the epidermal and dermal musculature of the posterior half of the affected fish. The parasite reaches the blood stream quickly through the lesions on the skin and the ciliates rapidly invade and proliferate in the skin and gills. Afterward, the parasite consume both host cells and body fluids and can spread to the internal organs in the absence of any additional pathogens such as secondary bacterial invaders (Azad et al., 2007). Tetrahymena corlissi is the causative agent of scuticociliatosis, parasitizes skin and muscle, and sometimes invades body cavities of freshwater fishes. It has a high potential for systemic invasion, destroying tissues that lead to high mortalities of the host. When the disease manifests, the initial clinical symptoms include dark coloration, excessive mucous production, loss of scales, hemorrhagic lesions and/or bleached spots on the skin, and dermal necrotic lesions that finally destroy tissues leading to high mortalities. This parasite feeds on the epithelium of the skin and grows large enough to be visible to the naked eye. Afterward, some dermal necrotic lesions coalesce to form brownish musky clinical manifestations (Harikrishnan et al., 2010). Similarly, some fungi can give rise to skin lesions when grown on skin cells (e.g., Saprolegnia) by invading the epidermis and the dermis.
4.4.4 The embryology of the skin immune system
While the structure, organization, and composition of fish skin have attracted the attention of researchers for a long time, information on skin development remains scarce. During the first half of the last century most studies on fish skin have been limited to morphological descriptions of structural diversity, usually in relation to the environment of the fish. With the introduction of the electron microscope, some authors have investigated the structure of fish skin (mainly epidermis), as well as scale structure and development. However, even at present there are very few studies dealing with fish skin development, and most available descriptions highlight features of adult skin. The origins of these skin layers of teleosts are still unknown and it is also surprising that the teleost dermis has not been well characterized in any species. One major developmental process of fish is the formation of the neural crest, which is found only in chordates. From this channel-like structure the neural tube is established (which is located between the epidermis and notochord). Cells of the neural crest migrate to different parts of the embryo, where they differentiate into diverse types of cells, like parts of the teeth, cartilage, or sensory cells of the skin. All vertebrates initially have the same type of skin. Only later does the skin develop fish scales, reptilian scales, bird feathers, or the hair, claws, and nails of mammals. The epidermis differentiates from an epidermogenic cell population of the embryonic ectoderm, along with neurogenic cell populations giving rise to the central nervous system and the neural crest. However, the dermis derives from the paraxial mesoderm (dermatome region), except for the head region, where it derives from the (ecto) mesenchyme of neural crest origin. These studies have been done in zebrafish. In embryos (at 90% epiboly) the epidermis consists of a single layer of more or less polarized cells covered by an external, “enveloping” layer, the periderm. The epidermal cells fuse at the midline approximately at 14-somite stage (Schmitz et al., 1998). The epidermis in embryos and young larvae is very thin (less than 10 mm thick). During week 1 of fish larval development, the epidermis is not differentiated in various layers yet, but by weeks 4–6 the thickness increases and various layers appear, depending upon the region of the body. In consequence, only transmission electron microscopical studies can provide an accurate morphological description of the early development of fish epidermis and dermis (Le Guellec et al., 2004). In very early developmental stages, the osmoregulation relies on the skin integument that is covered by ionocytes, in which the ion pumping activity takes place. Several types of pigment cells arise from the neural crest, including xanthophores, iridophores and melanocytes. This evolutionary achievement is linked with important features of fish skin: a stratified mucogenic epidermis and an alpha-keratogenic potential. Mucous cells differentiate early in development on the surface of epithelium and contain acid and neutral mucopolysaccharides. Primordial sensory buds are visible within the epithelia of the skin of the head in week 1 larvae, and become numerous during later larval development. Club cells, specialized epidermal cells that have an immune function, appear in the middle layer of the head and trunk epidermis on week 4 (Saadatfar et al., 2010).
The fish immune system is adapted to the fact that fish are free-living organisms from the embryonic stage of life in their aquatic environment. For this main reason, fish rely on their innate immune system for a long period of time, beginning at the early stages of embryogenesis. The barrier defenses and innate immune proteins play critical roles during the early-stage fish embryos prior to the development of functional organ systems. The innate immune proteins present in the yolk of embryos are of maternal origin. Early-stage teleostean embryos are dependent upon the adult female for the formation of the zona pellucida as an essential barrier defense, for their supply of nutrients, and for the innate immune proteins and antibodies that are transferred from the maternal circulation to the oocytes. Maternally derived hormones are also transferred, some of which (e.g., cortisol) are known to exert a suppressive action on some aspects of the immune defenses (Li and Leatherland, 2012). The adult skin develops from the ectoderm on the surface of the post-gastrulation embryo. Skin is a dynamic structure with proliferation in the basal layer, which is in contact with a basement membrane. The skin stem cells are located in the basal part of the epidermis and in specialized compartments of skin appendages. When cells finish differentiation, they cease proliferation and migrate upward, losing contact with the basement membrane. It is known that epidermis is constantly renewed, with a fine equilibrium between proliferation and differentiation. A key characteristic of this protective outer layer is that it is continually shed by new cells. Skin appendages develop from embryonic skin resulting from sequential epithelial-mesenchymal interactions and help with epidermal repair and regeneration. The teleost dermomyotome has been studied including primary myotome morphogenesis, the relationship between the primary myotome and the dermomyotome, as well as the differentiation of axial and appendicular muscles and dermis from the dermomyotome (comprehensively reviewed in Stellabotte and Devoto, 2007). In contrast to the considerable knowledge of skin patterning and epithelial appendage development in mammals and birds, little is known about the molecular control of skin patterning and scale morphogenesis in fish. The term “scale” in the fish literature is often used as a widespread term for all the hard, normally flattened, skeletal elements found in the skin of aquatic vertebrates. These include the scales of chondrichthyans (placoid scales), the scales of basal actinopterygians (ganoid scales), the bony scales of some actinopterygian taxa (dermal bony scales and scutes), and the scales of basal sarcopterygian taxa and most actinopterygian species (elasmoid scales). Although all these types of appendages are evolutionary linked as derivatives of a common ancestral type, they have a different structure (Sire and Akimenko, 2004). The establishment of the squamation pattern has been described in several teleost species. In all fish species studied so far, the scales appear very late in ontogeny, that is, several weeks post-fertilization, after metamorphosis, when the juveniles are already miniatures of the adults. In the zebrafish skin, fibroblasts, from which the scale-forming cells will differentiate, invade the primary dermal stroma only 20–26 days post-fertilization (Le Guellec et al., 2004), suggesting that fibroblast invasion (and further skin development) requires a specific state of differentiation of the collagenous stroma, and of the cells of the basal epidermal layer. Alizarin red staining of juvenile zebrafish has revealed that scale appearance is related to a combination of size and age.
4.5 Future directions and conclusions
Numerous laboratory models can be applied to better understand skin immunity but only some of them will be mentioned here. For example, the use of cell lines, which represent valuable biological tools for carrying out multiple experimental comparisons in pathology, carcinogenesis, and transgenics. Teleost fish cell lines have been developed from a broad range of tissues, including skin and most fish cell lines originated from normal tissues (Lakra et al., 2011). It has been shown that freshly harvested rainbow trout scales can be incorporated into fish skin cell cultures and, afterward, both cell types will proliferate and start to build connections with the other cell types. As the authors suggest, this seems to be the initial steps to generate “artificial skin” with two different cell types, potentially leading to the development of a three-dimensional skin (Rakers et al., 2011). Due to the phylogenetic position of fish in evolution and their atypical properties, fish skin offers a unique opportunity to study the origins of innate immune responses, which will be a very interesting research topic in the near future. Each fish species secretes a different repertoire of mucosal AMPs, which could be used by the pharmaceutical industry to identify novel drugs with antimicrobial functions, or new vaccine adjuvants, as part of inactivated vaccines or as antitumor agents. AMPs could be important when studying bacterial resistance to the drugs (antibiotics) used at present or as a potential source of antiviral compounds (Rajanbabu and Chen, 2011). Presently, genomic/transcriptomic approaches have propelled studies of different humoral immune components present in fish skin mucus, as well as their regulation after different stimuli, including natural or experimental infections. The available results confirm that complicated local signaling networks are present in the fish skin and these networks are involved in the immune response to different microorganisms. Studies of the skin transcriptome and proteome are scarce in fish, and have only recently begun. Omics-based studies will permit the discovery of new molecules not described by more traditional methods. Similarly, the strategies used by invasive pathogens to penetrate host cells or to evade killing will be studied, as well as molecular signatures related to host responses and host-pathogen interactions during infection. From a developmental standpoint of the skin, many interesting questions remain. For example, how is the transition achieved, in the early stages of skin development, from two cell layers toward the typical epidermis composed of three strata. Some interesting features of adult stem cells in fishes regarding the regeneration processes (e.g., skin damage) should be further investigated and then compared to the human integument. The study of fish skin reveals an abundance of problems that are also of major relevance to mammalian skin, highlighting potential uses of fish skin models in investigative dermatology. A heightened understanding of the biology of fish skin will enhance basic and clinical skin research, as well as comparative anatomy and physiology.