Introduction
The genus Lactobacillus belongs to the family of Lactobacteriaceae also known as lactic acid bacteria, a group of microorganisms that have been used for centuries in the preparation and processing of foods and beverages (Rose 1982; Kandler 1984; Chassy 1985, Chassy 1987).
These rod-shaped, anaerobic to micro-aerophilic, non-pathogenic bacteria, which produce lactic acid as their major end- product, are nowadays used in numerous fermentation processes, either alone or together with other organisms such as streptococci, pediococci, lactococci, leuconostocs and yeasts (Kilara & Treki 1984).
Particularly in dairy industry these bacteria are of vital importance. Fermented dairy products represent about 20% of the total economic value of fermented foods worldwide (Sharpe 1979). In addition, lactobacilli are used in the production and preservation of sausages and meat, in fermentation of olives and vegetables, in baking, and in the preparation of silage. To maintain products of high quality and to guarantee reproducibility of production processes, cultures of bacteria with selected and predictable properties are used as starters to inoculate food or feed. Some fermented milk products for many years are believed to have certain health promoting properties for humans.
This assumption was originally largely based upon Metchnikoff's theory (Metchnikoff 1908) that harmful effects of undesired bacteria can be overcome by establishing a new balance between intestinal bacteria, through ingestion of lactobacilli or fermented products made by these organisms. Research carried out during the last few decades has resulted in additional claims of health and/ or nutritional benefits for humans and animals associated with the consumption of fermented milk products.
Such claims include: control of intestinal infections, improved nutritional value of some foods, control of serum cholesterol levels, improvement of lactose metabolism, induction of a-specific and specific immune responses, and anti-carcinogenic activity (Fernandes et al. 1987; Gurr 1987; Perdigon et al. 1988; Gerritse et al. 1991a, b). Some of these - assumed - properties of certain Lactobacillus species have also focused interest on their use as probiotics to improve the growth potential of livestock.
Due to its great economic importance for the agro-feed sector and its alleged importance for human and animal health, research on selection and characterization of Lactobacillus strains, on metabolism, physiology and genetics of these organisms, and investigations aimed at the improvement of the characteristics of such strains have increased progressively over the last decade. Based on knowledge and methods applied to the development of genetic manipulation systems for other microbes, in particular Escherichia coli, Bacillus subtilis and Lactococcus, rapid progress has been made in developing the methodology for genetic manipulation of lactobacilli by recombinant DNA techniques.
Initially, research was focused on the characterization of Lactobacillus DNA by cloning and expression of DNA fragments in E. coli, and on the isolation and characterization of phages and plasmid DNA molecules. Aided by the development of a reproducible system to transform Lactobacillus strains (Chassy & Flickinger 1987; Aukrust & Nes 1988; Luchansky et al., 1988), methods became available to (re)introduce DNA into these organisms.
This has prompted studies of more fundamental processes like DNA replication and control of gene expression, as well as studies aimed at the improvement of specific properties of lactobacilli. As a result of the efforts carried out in a number of laboratories, Lactobacillus research has come of age. Methods for the introduction and stable maintenance of DNA into Lactobacillus are routine now and can be applied to almost any Lactobacillus species. Both broad host-range and narrow host-range multi-copy plasmid vectors based on a variety of replicons have become available for the introduction and expression of homologous and heterologous genes. Finally, methods have been developed to insert genes at specific sites of the chromosome allowing genes to be mutated at will. No attempts will be made in this review to be exhaustive with respect to the genetics of lactobacilli. In this review the state of the art will be presented of our knowledge concerning plasmid structure and plasmid replication. Secondly, a description will be given of our present knowledge of gene structure and gene expression of lactobacilli.
For further details on the chromosomal organization of the Lactobacillus genome, on the genetics of phages and phage resistance, and gene-transfer systems the reader is referred to recent review articles (Chassy & Murphy 1993; Le Bourgeois et al. 1993; Mercenier et al. 1993; Pouwels et al. 1992).
Plasmids
Occurrence
Plasmids have been found in many Lactobacillus spp. since they were first discovered in 1976 by Chassy and co-workers (Chassy et al. 1976; Hofer 1977; Chassy et al., 1978; Smiley & Fryder 1978; Ishiwa & Iwata 1980; Klaenhammer & Sutherland 1980; Vescovo et al. 1981; Vescovo et al. 1982; Morelli et al. 1983a, b; Klaenhammer 1984; Nes 1984; West & Warner 1985; Jewell & Collins-Thompson 1989; Rinckel & Savage 1990). Several studies concerning plasmid contents of lactobacilli isolated from plant material (Daeschel et al. 1987), meat (Ahrn6 et al. 1989; Ahn & Stiles 1990), silage (Hill & Hill 1986), sour dough (Spicher & L6nner 1985; L6nner et al. 1990) and the gastro-intestinal tract (Klaenhammer & Sutherland 1980; Vescovo et al. 1982; Lin & Savage 1985) have been reported.
From these studies it has become clear that many, but not all species, harbour one or more plasmids. Only a fraction of L. plantarum strains isolated from different sources was reported to contain plasmids (Klaenhammer 1984; Nes 1984; West & Warner 1985; Hill & Hill 1986; Von Hysby & Nes 1986; Mayo et al. 1989). Differences in plasmid content of Lactobacillus strains reported by different investigators, may in part be explained by differences in plasmid extraction methods. However, also differences in source of strains can account for such differences. In contrast with most of the aforementioned studies, Ruiz-Barba et al. (Ruiz-Barba et al. 1991) detected a variety of plasmids in each of the 35 L. plantarum strains from olives and many of the plasmids were found to be of considerably higher molecular weight than those previously observed.
In Lactobacillus strains isolated from the gastro-intestinal tract from chickens and mice, plasmids were detected in a minor fraction of strains analyzed (Lin & Savage 1985; Posno, pers. comm.). Plasmids are also rarely found in L. bulgaricus strains (Chassy, pers. comm.). L. bulgaricus strain 10 (Culture Collection at North Carolina State University) and L. bulgaricus strain M-878 (Meiji Institute of Health Science, Japan) appear to form the exception (Chagneaud et al. 1992; Sasaki, pers. comm.).
Plasmids with variable degrees of sequence homology, as revealed by DNA-DNA hybridization and/or nucleotide sequence analysis, are found in several species (Bringel et al. 1989; Josson et al., 1989; Vogel et al. 1991; Leer et al. 1992), suggesting that horizontal transfer of plasmids and/or recombination between plasmids are rather frequent events.
Although their ubiquitous presence is well established, little is known about the function of plasmids. Unlike in lactococci, where the occurrence on plasmids of genes involved in essential functions like proteolysis (Kok 1990) or sugar metabolism (Gasson 1990), and other traits like phage resistance and conjugal transfer (Sanders et al. 1986; McKay & Baldwin 1984; de Vos et al. 1984) and bacteriocin production (Klaenhammer 1993) is quite common, few such plasmid-borne traits have been found in lactobacilli.
During the last few years extensive research has been carried out to link plasmid content with specific bacterial characteristics. Except for cases where could be correlated with such phenotypical properties as drug resistance (Ishiwa & Iwata 1980; Vescovo et al. 1982; Morelli et al. 1983a, b; Axelsson et al. 1988), N-acetyl-glucosamine and slow acid formation (Smiley & Fryder 1978), glucidic metabolism (Liu et al. 1988), carbohydrate metabolism (Chassy et al. 1978; Chassy & Rokaw 1981; ShimizuKadota 1987; Kanatani et al. 1992), aminoacid metabolism (Shay et al. 1988), bacteriocin production and immunity (Muriana & Klaenhammer 1987; Schillinger & Lficke 1989; Van der Vossen et al., in preparation) or slime production (Ahrn6 et al. 1989), most plasmids in Lactobacillus, in particular small plasmids, are cryptic.
Segregational stability
Most indigenous Lactobacillus plasmids are segregationally stable. A number of strains have already been propagated under experimental conditions for many years without noticeable changes in plasmid content. Some exceptions have, however, been found. For example, maltose utilization which is a plasmid-borne trait in some lactobacilli present in meat, was found to be unstably inherited (Liu et al. 1988). Three consecutive transfers in the presence of acriflavine resulted in an almost complete loss of the plasmid. Similar phenomena where observed for plasmid-linked galactose utilization markers from a strain of L. acidophilus (Kanatani et al. 1992) and plasmid-borne lactose markers (Chassy et al. 1978). In these cases the markers were found to be present on large plasmids (40-80 MDa). The relative instability of large plasmids contrasts that of the small cryptic plasmids.
A number of plasmids found in strains of e.g.L, plantarum or L. pentosus cannot be eliminated by cultivation of the bacteria at sublethal temperatures in the presence of acriflavine or novobiocin (Bringel et al. 1989; Leer, unpublished observations), conditions that are effective in curing of most other bacterial strains of endogenous plasmids. The presence of these small plasmids might confer a selective advantage over strains lacking them under conditions used outside the laboratory, although they appear not to carry essential genes.
Plasmid structure
The nucleotide sequence of eight small cryptic plasmids from Lactobacillus spp. has been determined. Plasmids pLB4 (Bates & Gilbert 1989), pC30il (Skaugen 1989), pLPI (Bouia et al. 1989), p8014-2 (Leer et al. 1992) and pAl (Vujcic & Topisirovic 1993) are from L. plantarum, p353-2 (Leer et al. 1992) from L. pentosus, pLJ1 (Takiguchi et al. 1989) from L. helveticus and pLAB1000 (Josson et al. 1990) from L. hilgardii. The organization of the plasmids, which vary in size from 1.9-3.5 kb, is very similar. All plasmids, except pLJ1, code for a protein showing similarities to the replication proteins (Rep) of plasmids from Gram-positive bacteria. The involvement of this protein in replication has been demonstrated by in trans complementation assays (Bringel et al. 1989; Josson et al. 1990).
Based on the functionality of these proteins as replication proteins and their structural similarity to replication proteins from other plasmids, it was assumed that small plasmids from Lactobacillus spp. replicate by a mechanism of Rolling Circle Replication (RCR). Direct proof for this hypothesis has been obtained for pLAB1000 and p353-2, by showing the accumulation of single-stranded DNA replication intermediates (Josson et al. 1990; Leer et al. 1992). The minimal replication region has been determined for pLAB1000 in Bacillus, Enterococcus and Lactobacillus strains as being 1.5 kb (Josson et al. 1990). Based on the similarity of the structure of pLAB1000 and other plasmids from Lactobacillus spp. with that of other RCR plasmids from Grampositive bacteria, it seems fair to assume that the minimal replication region for all other Lactobacillus plasmids is comparable in size.
Replication protein
The replication proteins of RCR plasmids have nicking-closing activity at a specific site of the plusDNA strand, called plus-origin of replication, dso (double-stranded DNA origin, previously called ori (+)) as was first demonstrated for pT181 (Koepsel et al. 1987).
RCR plasmids have been grouped into four families based on structural similarities of the replication proteins and cognate dso (Gruss & Ehrlich 1989; Bron 1990; Ilyina & Koonin 1992), exemplified by pT181 (Khan & Novick 1983), pUBll0 and pC194 (McKenzie et al. 1986, 1987; Horinouchi & Weisblum 1982b), pLS1 and pE194 (Lacks et al. 1986; Horinouchi & Weisblum 1982a) and pSN2 (Khan & Novick 1982). All plasmids from Lactobacillus spp. either belong to the second or third category, as judged by this criterium (Table 1).
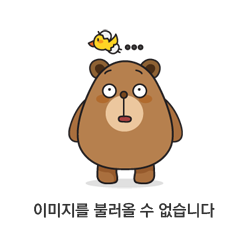
Although Rep proteins and dso sequences from pLAB1000 and pUB110 display extensive similarity, no transcomplementation is observed (Josson et al. 1990), indicating that Rep proteins show strict specificity for their target nicking site, even towards members of the same family. A similar phenomenon was observed for plasmids from the pT181 family (Iordanescu & Projan 1988). The Rep proteins from pAl and pLB4 are similar to pE194 and other members of the pLS1 family of plasmids. Plasmid pLJ1 is a 3.3 kb plasmid containing one open reading frame (ORF) which could code for a protein of 41 kDa, presumed to be the replication protein. No sequence similarity with other known (replication) proteins has been found, suggesting that the Rep protein of pLJ1 might rep- resent a new class of RCR replication proteins. Alternatively, pLJ1 might replicate by a different mechanism. A comparison of the structure of replication proteins of a variety of plasmids from Staphylococcus, Bacillus, Streptococcus and Lactobacillus indicates that the Rep proteins from Lactobacillus plasmids pLAB1000, pC30il, pLP1, p8014-2 and p353-2 form a cluster that is closely related to the Rep protein of pUB110 but more distantly related to that of pC194 (Alonso pers. comm.).
Mobilization functions
Plasmids pLB4 and pLAB1000 carry a gene coding for a 42-46 kDa protein which is presumably involved in plasmid mobilization. The protein encoded by pLAB1000 was shown not to influence DNA replication in Lactobacillus, Bacillus and Staphylococcus strains (Josson et al. 1990). The mob gene product is thought, by analogy with similar proteins from pE194, pT181 and pUB110, to fuse plasmids at a specific co-integration site called, RSa, allowing mobilization of non-conjugative plasmids. An RS A site showing considerable similarity to that present in pE194 and pT181, has been found in plasmids pLB4 and pLAB1000 (Bates & Gilbert 1989; Josson et al. 1990), as well as in pAl (Vujcic & Topisirovic 1993). interestingly, pAl does not code for a Mob protein but contains an ORF which could code for a protein of 103 amino acids showing extensive similarity to the N-terminal region of Mob proteins from other plasmids. This truncated mob gene is probably not expressed as no expression signals were found 5' to the gene and no protein of the expected size could be demonstrated when the plasmid was expressed in vitro (Vujcic & Topisirovic 1993). The RS A site in pLAB1000 overlaps the promoter of the mob gene as was found for plasmid pT181 (Gennaro et al. 1987).
Control of DNA replication
Plasmid DNA replication is regulated through negative control mechanisms by plasmid-borne repressors. These trans-acting repressors bind to plasmidspecific targets, controlling plasmid replication in an indirect way (Novick 1987; Thomas 1988). For many plasmids antisense RNA (also called countertranscript RNA or CT-RNA) has been implicated in the mechanism of control of plasmid DNA replication. These CT-RNAs, which are complementary to the 5' end of Rep protein encoding RNA, interfere with the expression of the rep gene, by a mechanism of transcription attenuation (Novick et al. 1989) or by inhibition of translation initiation (Alonso & Taylor 1987; Maciag et al. 1988; Del Solar & Espinosa 1992). In only one of the plasmids from Lactobacillus spp., pLB4 from L. plantarum, has a gene been found which might encode a repressor protein.
The protein shows similarity to repressors encoded by members of the pLS1 family of plasmids. By analogy with pLS1, the pLB4 repressor might repress the synthesis of rep RNA and, in addition, control its own synthesis (Del Solar & Espinosa 1992). These authors suggested that control of DNA replication in pLB4 is also exerted at the level of translation initiation by a CT-RNA which sequesters the Shine-Dalgarno sequence of rep RNA (Shine & Dalgarno 1974). Plasmid p353-2 of L. pentosus codes for two CTRNAs of ~ 75 and ~ 250 nucleotides transcribed from the region encoding the 5' end of the rep gene and in the opposite direction (Pouwels et al. 1993). CT-RNA negatively controls plasmids DNA replication. Control of plasmid replication is exerted by a mechanism involving transcription attenuation of rep RNA at a site just in front of the rep gene. In the presence of CT-RNA (wild-type plasmid) more than 90% of transcription initiated at an upstream promoter is prematurely terminated at this attenuator, whereas in the absence of CT-RNA nearly all transcripts reach a size corresponding to that of the rep gene (Fig. 1). A computer-assisted analysis of RNA secondary structures indicates the presence near the 5' end of rep RNA of a sequence which can hybridize to part of the attenuator sequence, precluding the formation of the attenuator stem-loop structure. Secondary structure predictions also suggest that CT-RNA induces a conformational change of the 5' untranslated region of rep RNA, resulting in the formation of the transcription terminator (Pouwels et al. 1993). The dissimilarity of the replication proteins but similarity of the organization of the replication regulatory region and mode of regulation of DNA replication of p353-2 and members of the pT181 family of plasmids is of importance with respect to the evolutionary relationship between the two plasmids. In contrast to p353-2 and pT181, but similar to plasmid pLS1, control of replication of pC194 and pUB110 takes place post-transcriptionally and might involve sequestration of the translation-initiation signals (Alonso & Taylor 1987; Maciag et al. 1988). These data indicate that the mode of control of DNA replication is different for different members of the pUB110 family of plasmids. Apparently, replication proteins and replication control region are found in different combinations, presumably as a result of horizontal transfer of DNA elements (Projan & Novick 1988; Gruss & Ehrlich 1989; Ilyina & Koonin 1992; Pouwels et al. 1993).
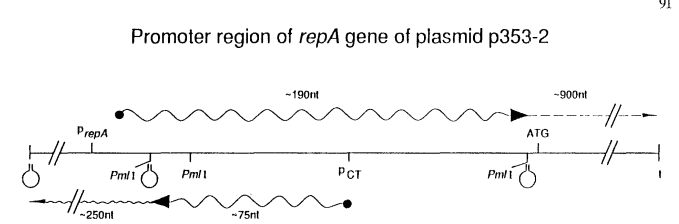
Fig. 1. Promoter region of repA gene of plasmid p353-2 from L. pentosus, repA RNA synthesis is controlled by an antisense RNA (CT-RNA) which induces the formation of a transcription attenuator, just in front of the ATG codon. As a result, more than 90% of all transcripts initiated at the promoter of repA are arrested at the attenuator. Deletion of the promoter of CT-RNA (Pm/I fragments) results in readthrough at the attenuator which is accompanied by a 5-10-fold increase of the plasmid copy number.
'Genetics of lactobacilli' 카테고리의 다른 글
Genetics of lactobacilli: plasmids and gene expression - Plasmid vectors(진행중) (0) | 2024.11.16 |
---|