Introduction
The intestinal epithelium is a single cell layer protecting the organism against harmful agents in the lumen, and at the same time it is a site for nutrient, water and ion uptake. Integrity of the barrier and uptake mechanisms is crucial for the health and growth of the animal.
However, many internal and external factors can influence the epithelium in both harmful and beneficial ways. The focus of the present thesis is to elucidate how factors such as pathogens, diet and developmental stage affect the physiological function of the intestinal epithelium in salmonids.
The intestinal epithelium
Intestinal morphology
The main functions for the gastrointestinal (GI) tract are: to store food and water, to process the ingested food and water; to absorb water, osmolytes and nutrients from the external medium, and to excrete waste. In vertebrates, the GI canal is constituted of several distinct regions that differ in morphology and histology as well as in physiological functions. Following prey capture and manipulation by teeth or other parts of the oral cavity, the esophagus transports the food to the stomach.
The stomach is absent in some vertebrates, such as some species of fish, in which the intestine directly follows the esophagus. Stomach secretions typically contain proteolytic enzymes as well as hydrochloric acid. After mixing and processing in the stomach, the bolus is emptied into the intestine where absorption occurs. There are large differences in the morphology and physiology of the intestinal regions between the vertebrate groups and between different feeding strategies within the same vertebrate group.
Mammals have several distinct regions of the intestine, whereas other vertebrate groups such as the cyclostomes only have one (Nilsson 1983). In fish, intestines vary in length from 0.4 to >38 times the body length. The percentage plant material in the diet is the major determining factor for intestinal length, where intestines of herbivorous fish generally are longer than those of carnivorous fish (Buddington et al. 1997; Clements and Raubenheimer 2006). Teleost intestines commonly have two regions, in this thesis referred to as the anterior and the posterior regions, separated by the ileocolonic junction (Clements and Raubenheimer 2006).
At the anterior end of the anterior intestinal region, many fish species have numerous pyloric caeca which extend the surface area (Clements and Raubenheimer 2006; Veillette et al. 2005).
Functionally, the anterior region and the pyloric caeca are the primary sites for nutrient uptake (Nordrum et al. 2000), whereas the posterior region has less nutrient absorptive capacity and more phagocytotic activity (Buddington and Diamond 1987; Ezeasor and Stokoe 1981)
The pinocytosis of proteins by the posterior region has been suggested to have nutritional importance (Clements and Raubenheimer 2006).
Layers of the intestinal wall
Despite the many specialized regions of the GI tract, cross-sectional tissue organization remains fairly similar throughout the intestines of vertebrates. The vertebrate intestine consists of several histologically distinct tissue layers with correspondingly distinct functions.
Lining the lumen is the epithelium, the barrier between the exterior and interior medium (Figure 1), which is attached to the connective tissue layer of the basement membrane. The surface area of the mammalian intestinal epithelium is expanded through fingerlike villi. Fish intestinal epithelia are also expanded through folding, but lack the typical crypts of the mammalian villi.
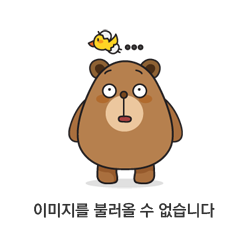
Figure 1. Fluorescence microscopy image using triple filters (FITC, DAPI and Texas Red). Cross sectional view of the anterior intestine of rainbow trout. Layers of the intestinal wall: 1. Lumen 2. Goblet cells (black) 3. Enterocytes 4. Basement membrane 5. Lamina Propria 6. Submucosa 7. Circular and longitudinal muscle layers 8. Serosal layer removed
The term mucosal folds will hence be used when referring to the fish epithelial folding. The epithelium, together with the underlying lamina propria constitutes the mucosa. Adjacent to the lamina propria is the connective and contractile muscularis mucosa which separates the lamina propria from the submucosa.
Within the submucosal layer, the submucosal nerve plexus is found. Further away from the lumen, the circular muscle layer is followed by the myenteric nerve plexus and the longitudinal muscle layer. The perimeter of the intestine is lined by the serosa, a connective tissue layer attached to the mesenteric tissue.
The intestinal circulation
In addition to the regular gas exchange, nutrient and waste transport that occur in all tissues, the intestinal circulation also performs the task of removing absorbed substances from the epithelium of vertebrates. Arterioles and venules extend into the lamina propria of the mucosal fold tips in close proximity to the epithelium.
The veins leaving the intestine collect in the portal vein leading to the liver for nutrient metabolism, detoxification and immune functions (Guyton and Hall 2000).
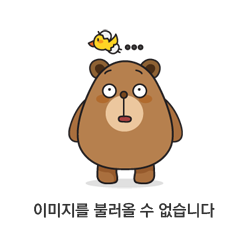
The close contact between arterioles and venules in the villi in mammals has been shown to function as a counter-current gas exchanger that reduces oxygen content in the villi tips (Haglund 1994). If a similar countercurrent mechanism is functional in fish is not known.
Fish differ from other vertebrates in that they lack a true lymph system and instead have a secondary circulation.
This circulatory system is connected to arterioles but have high resistance sphincters that reduce entry of red blood cells. The function of the secondary circulation is not well known, but it has been speculated that it may aid in osmoregulation and possibly be a precursor for the lymphatic system (Clements and Raubenheimer 2006).
Epithelial morphology
The epithelial layer consists mainly of absorptive columnar cells, referred to as enterocytes, with the inclusion of mucus-secreting goblet cells and endocrine cells (Figure 1). On the apical (luminal) surface of the enterocytes are numerous extensions called microvilli (Figure 2), and the whole apical surface of the epithelium is referred to as the brush border membrane (BBM).
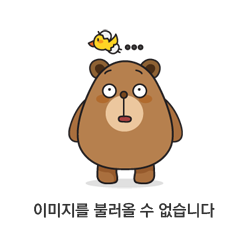
Figure 2. Transmission electron micrograph of the anterior intestinal brush border membrane from rainbow trout. The intestinal segment was mounted into Ussing chambers for 150 minutes of regular control experiment, before fixation. Visible structures are: microvilli (MV), tight junctions (TJ), desmosomes (DS) and mitochondria (MC). Enlargement ×20.000. (TEM image: Sundell unpublished).
The microvilli greatly extend the surface area of the apical membrane which increases the area for absorption and membrane-bound digestive and absorptive enzymes (Clements and Raubenheimer 2006).
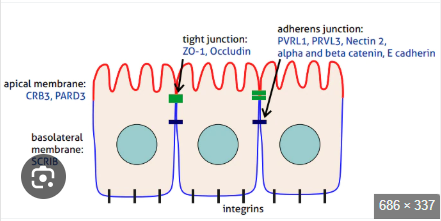
Adjacent enterocytes are joined together at the apical end of the lateral surface by junctional complexes.
The junctional complexes consist of anchoring adhesion belts and desmosomes, and located closest to the lumen are the occluding tight junctions (TJ). The limit for the paracellular permeability is set by the occluding TJ, which are vital for the function of the epithelial monolayer.
The tight junctional complexes are chains of transmembrane junctional proteins forming continuous seals between the adjoining epithelial cells (Anderson 2001). By the use of freeze-fracture electron microscopy, TJ complexes have been described as Ø10 nm particles spaced at 18 nm (center to center). Aqueous pores are thought to cross the TJ strands with different permeability in different tissues and regions of the intestine. The pores of the intestinal TJs display positive cation selectivity.
More than one TJ pore size may be found simultaneously in the same tissue. The proteins of the TJ complexes can be divided into three functional and morphological groups:
1. The extracellular proteins that span the paracellular gap and form the actual paracellular barrier. These consist of occludin and claudins. While occludin was originally thought to be the major component of the extracellular TJ complex, several claudins are now the prime candidates (Schneeberger and Lynch 2004). Claudins are, like occludin, tetraspan membrane proteins with two loops, but unlike the similarly sized loops of occludin, one of the claudin loops is shorter than the other. Over- and under-expression of different claudins influences cation permeability through epithelia, indicating that claudins are responsible for the previously observed cation selectivity through the TJs (Johnson 2005). Long term regulation of TJ permeability is considered to include changes in the amount of TJ proteins (Johnson 2005).
2. TJ plaque proteins connect the transmembrane proteins to the actin cytoskeleton and are thought to be responsible for the rapid regulation of TJ permeability. The cytosolic plaque proteins consist of several families with the major family being the zona occludens proteins (ZO 1-3).
3. Cytosolic and nuclear proteins which interact with TJ plaque proteins to regulate among other things the paracellular permeability. Together, these proteins constitute the regulated physical barrier connecting the epithelial cells. The regions of the intestinal tract differ in their luminal content and may thus need different TJ permeabilities in the different regions. Indeed, the paracellular permeability correspondingly differs between intestinal regions in mammals (Baumgart and Dignass 2002) as well as in fish (Schep et al. 1997).
Epithelial Barrier function
Extrinsic barrier
Commensal bacteria inhabit every ecological niche of the intestinal lumen and bacterial counts can reach 10^12 cells g-1 mammalian luminal content. In humans, bacterial cells outnumber the human cells (Mueller and Macpherson 2006) whereas the bacterial concentration in fish intestinal luminal content is lower, ranging from 10^5 to 10^8 cells g-1 (Cahill 1990; Ringø et al. 1995).
Under normal circumstances, the bacteria remain harmless in the lumen, and may even facilitate the digestion or production of nutrients otherwise inaccessible to the host (Ringø et al. 1992). Gut bacteria benefit from the symbiosis by the intestinal environment and available nutrients (Ismail and Hooper 2005).
Commensal bacteria may also protect the host from pathogenic bacteria by means of ecological competition for resources or through direct inhibition by the secretion of peptide bacteriocins, H2 O2 or organic acids such as lactic acid (Montagne et al. 2004; Ringø and Gatesoupe 1998).
In fish, bacteria isolated from the gut inhibit the growth in vitro of bacterial fish pathogens including Aeromonas salmonicida (Irianto and Austin 2002; Jöborn et al. 1999; Robertson et al. 2000). In vivo, diets containing lactic acid bacteria may confer some resistance against pathogenic bacteria.
Juvenile Atlantic cod (Gadus morhua) fed a diet containing lactic acid bacteria were more resistant to infection, an effect speculated to be caused by ecological competition in the gut (Gildberg et al. 1997). Atlantic salmon and rainbow trout were more resistant during a disease challenge test with A. salmonicida (Robertson et al. 2000).
Besides the ecological competition as an effect of probiotic bacteria, stimulation of the gut immune system by the endogenous bacterial flora has also been suggested as a protective mechanism (Irianto and Austin 2002). Some studies have shown a lack of protective effect of probiotic lactic acid bacteria (Gildberg et al. 1995).
In the epithelium, goblet cells secrete mucus (Figure 1), a viscous fluid containing mucin glycoproteins, to the apical surface of the epithelium. The mucus physically removes bacteria from the BBM by the constant flow away from the BBM and by receptor sites on the glycoproteins where bacterial adhesion factors attach (Maxson et al. 1994; Montagne et al. 2004).
Deletion of mucus secretion reduces the barrier function of this layer (Maxson et al. 1994). Mucus may also be a homing cue for pathogenic bacteria in order to locate epithelia. Indeed, the fish pathogen Vibrio anguillarium has positive chemotaxis towards mucus from fish intestinal epithelia (Larsen et al. 2001).
Intrinsic barrier
The intestinal tract is an entry point for infection by pathogenic organisms in vertebrates. During the 1960’s, Wolochow and coworkers investigated the intestinal epithelial barrier in rats (Rattus norvegicus) and concluded that bacteria instilled intra-intestinally later could be isolated from the lymph-nodes and blood.
This process was termed translocation (Wolochow et al. 1966) and later defined as the transport of viable bacteria from the lumen to extra intestinal sites (Berg 1995), but is presently also used to describe transport of bacteria across the intestinal epithelium only.
In the present thesis, the term bacterial translocation is used when referring to transport across the entire intestinal wall with all its layers of barriers. The intrinsic barrier function consists of the physical epithelial wall working in concert with other mechanisms to prevent organisms to enter the host tissues. Starting from the luminal side, the epithelial monolayer with tight junctional complexes creates the primary physical barrier between the lumen and lamina propria (Clayburgh et al. 2004). As described above, tight junctional pores (~7- to 15-Å Ø) are too small for bacteria (~1 µm or 10.000 Å) to pass. However, some bacterial toxins may still be able to cross the physical barrier (Dalmo and Bogwald 1996; Popoff 2005).
Immunological barrier
The mucosal immune system (or gut-associated lymphoid tissue, GALT) of the jawed vertebrates comprises two functional groups, the innate and the adaptive immune system (Forchielli and Walker 2005).
The innate immune system creates the first line of defense with rapid response and clearance of pathogens (Collier-Hyams and Neish 2005; Muller et al. 2005), whereas the adaptive immune system is slower but produces specific responses to the pathogen and maintains the specific response for a longer time period (Cheroutre and Madakamutil 2005; Nejdfors 2000).
In fish, the innate immune system is suggested to have higher importance than in mammals, possibly because the specific defense is slower in ectothermic vertebrates (Ellis 2001; Magnadottir 2006). Antibody production in salmonids takes weeks while bacterial infections can kill in days, which demonstrates that the innate immune system is of high importance for salmonids (Ellis 2001).
Total exclusion of microorganisms by the epithelium is probably not possible. Some passage of bacteria across the epithelium is inevitable and may even, at least in mammals, be actively promoted by the host (Kucharzik et al. 2000). Specialized regions of the mammalian epithelium called Peyer’s patches (also often referred to as the follicle associated epithelium; Peyer’s patches will be used in the present thesis) continuously sample gut microbiota in order to prime the immune responses and antibody production towards the antigens present (Acheson and Luccioli 2004).
Among the epithelial cells of the Peyer’s patches are M-cells specialized for antigen sampling. These cells have high phagocytotic and transcytotic activity of luminal antigen from the apical to the basolateral side of the M-cells.
There, intraepithelial and lamina propria lymphocytes and T-cells sample the antigens and induce the appropriate immune responses such as production of cytokines and specific antibodies (Kucharzik et al. 2000) and antigen recognition retention in memory cells (Cheroutre and Madakamutil 2005).
Birds have a system for antigen sampling which is similar to the mammalian Peyer’s patches (Pohlmeyer et al. 2005), whereas it is unclear if the structures and functions exists in reptiles or amphibians (Hart et al. 1988). It has been suggested that Peyer’s patches and M-cells are absent in the fishes (Buddington et al. 1997; Rombout 1998). However, the American paddlefish (Polyodon spathula Walbaum) has mucosal lymphoid follicles which form structures resembling the mammalian Peyer’s patches (Fänge 1984).
The function of the Peyer’s patches-like structures of the paddlefish has not been investigated, but histological data suggests a function similar to the Peyer’s patches of mammals (Petrie and Peterman 2005). The spiral valve of the elasmobranch intestine has mucosal lymphocyte accumulations which have a structure similar to Peyer’s patches. The function of these structures is not determined (Hart et al. 1988).
The teleost mucosal immune system is more diffuse, consisting of antigen processing macrophages in the epithelium and lamina propria. The posterior intestine is considered to have more intraepithelial macrophages than the anterior region.
These cells are suggested to function as antigen presenting cells (Buddington et al. 1997; Pettersen 2003). Intestinal B-cells and T-cells have been found in teleosts (Hart et al. 1988; Rombout 1998; Zapata et al. 2006), but surprisingly, are lacking sometimes (Wermenstam and Pilstrom 2001).
Whether the antigen sampling function of the mammalian M-cells is performed by other epithelial cell types in fish, such as the enterocytes, is suggested but still poorly understood (Hart et al. 1988; Rombout 1998).
Transport across the epithelium has been demonstrated in salmonids for luminal macromolecules (Brudeseth and Evensen 1995; Georgopoulou et al. 1988; O’Donnell et al. 1994) as well as bacterial cells (Hart et al. 1988; Ringø 2006; Vigneulle and Laurencin 1991).
The higher phagocytotic activity of the posterior region in comparison to the anterior region of fish intestines, as described below, has been suggested to include an antigen sampling function by transferring antigen from the lumen to macrophages and lymphoid cells in the epithelium and the lamina propria (Hart et al. 1988; Rombout 1998).
Functionally, antigens presented orally can activate immune functions and induce production of specific antibodies and storage of antigen by memory cells (Georgopoulou and Vernier 1986; Nikoskelainen et al. 2003; Rombout 1998). Anal intubation of inactivated pathogenic bacteria in carp (Cyprinus carpio) has been shown to induce high levels of serum antibodies, as well as having a protective effect in subsequent challenge tests (Ellis 1995). The protection in those challenge tests were comparable to the protection after injection of the antigen, demonstrating that the carp posterior intestine has effective antigen sampling and presenting functions (Ellis 1995).
Lipopolysaccharide (LPS) is a constituent of the cell surface of gram-negative bacteria (Schletter et al. 1995). As many gram-negative bacteria are pathogenic to vertebrates, LPS is used by animals for early detection of infection by gramnegative bacteria.
LPS detection systems thus have a protective function as a warning system, the importance of which has been demonstrated by animals with dysfunctional LPS detection systems; as they are highly sensitive to infections by gram-negative bacteria (Schletter et al. 1995).
However, if the host immune response is too powerful, the animal can be harmed by the inflammation (Iliev et al. 2005; Munford 2005; Schletter et al. 1995). The innate immune system of teleosts, like that of other vertebrates, uses toll like receptors (TLR) to specifically detect pathogens (Plouffe et al. 2005). LPS is a potent inducer of the fish innate immune system through TLR activation (Iliev et al. 2005).
Mechanisms of bacterial translocation
In order to infect the host, bacteria first have to cross the multiple barriers of the intestinal wall. After growth and adhesion to the BBM, the epithelium has to be crossed.
Transcytosis, where bacterial cells are phagocytosed at the apical surface and exocytosed at the basolateral side of the epithelium is suggested to be the dominant route for translocation in mammals (Cossart and Sansonetti 2004), although paracellular translocation has also been suggested especially in areas with a damaged epithelium (Baumgart and Dignass 2002; Berg 1995; Nadler and Ford 2000).
Several mammalian bacterial pathogens utilize Mcells for epithelial passage (Kucharzik et al. 2000), since bacteria from many species are transcytosed without inactivation of the pathogen. An important virulence factor for these pathogens then becomes the ability to avoid or survive phagocytosis by the immune cells (Cossart and Sansonetti 2004).
Transcytosis can also occur through cells that may not normally phagocytose bacteria. A bacterial virulence factor for injecting substances into eukaryotic cells is called the type III secretion system (TTSS).
TTSSs are pore-like protein-structures across the bacterial envelope that include protruding needle-like structures which are able to penetrate host cell membranes (Buttner and Bonas 2003; Hueck 1998). When the bacteria come in close contact to the eukaryotic cell, the TTSS extend through the cell membrane of the host and substances can be injected directly into the cytoplasm (Hueck 1998).
The injected substances are proteins that may be necessary for infection to occur. Functions of the injected substances include host cell membrane-bound receptors for the bacteria (Nougayrede et al. 2003), inhibition of phagocytosis (Anderson and Schneewind 1999; Burr et al. 2005; Hueck 1998), cytotoxic and apoptosis-inducing effects (Hueck 1998), killing of neutrophils by necrosis (Mecsas and Strauss 1996) and disruption of the actin cytoskeleton (Lesnick and Guiney 2001).
In mammals, several species of gram-negative bacteria use contact dependent type III secretion of toxins for disruption of the intestinal epithelial barrier (McNamara et al. 2001; Parsot 2005), and activation of epithelial phagocytosis (Cossart and Sansonetti 2004; Guiney 2005; Mecsas and Strauss 1996; Parsot 2005; Popoff 2005).
'The Intestinal Epithelium of Salmonids' 카테고리의 다른 글
The Intestinal Epithelium of Salmonids - Transepithelial transport mechanisms (0) | 2024.12.01 |
---|