INTRODUCTION
The group of bacterial protein toxins termed pore-forming toxins (PFT) belongs to the family of toxins called membrane-damaging toxins (MDT) which possess the ability to damage or disrupt the integrity of the plasma membrane of various eukaryotic cells (impairment of osmotic balance) reflected by cell swelling and subsequently cell lysis (Bhakdi and Tranum-Jensen 1988; Menestrina and Vécsey-Semjén 1999; van der Goot 2001; Alouf 2001).
Many toxins of the MDT family (also known as cytolysins or cytolytic toxins) are important virulence and pathogenicity factors of various Gram-positive and Gram-negative bacteria. A wide range of information on these toxins may be found in recent textbooks (Alouf and Freer 1999; Aktories and Just 2000; van der Goot 2001; Salyers and Whitt 2002).
MDT were first identified by virtue of their lytic action on human or other animal species erythrocytes and thereby were designated hemolysins. This denomination which is still used in the literature is restrictive and somewhat inappropriate. It became clear from an early stage that many if not all hemolysins acted on cells other than erythrocytes and also caused tissue damage and even death for many of them in experimental animals. On the other hand, a number of MDT proved inactive on erythrocytes while they damaged other cells such as leukocytes (leukocidins). Therefore, the terms cytolysins or cytolytic toxins are preferable.
FUNCTIONAL CLASSIFICATION OF MEMBRANE-DAMAGING TOXINS
Three types of MDT can be distinguished, based on their mechanism of action against target cell membranes: (a) pore-forming toxins (cytolysins) which create channels (pores) through the plasma membrane (7–9 nm) of various human and/or animal cells; (b) enzymically active cytolysins which degrade the cell-membrane phospholipid bilayer; (c) tensio-active cytolysins which solubilize certain cell-membrane constituents by a detergent-like action (Bernheimer and Rudy 1986).
To date, the MDT family comprises 117 toxins (c. 35 % of the whole repertoire of 337 bacterial protein toxins so far known). In this respect, MDT constitute the most important family of bacterial toxins. The PFT group comprises 81 proteins while the enzymic and tensio-active cytolysin groups comprise 26 and 10 proteins, respectively. In the present review we will briefly describe the major genomic, molecular and structural features of the pore-forming toxins. The description of the biological, pathophysiological and clinical aspects of this large group of toxins are beyond the scope of this review. We refer the reader to the recent textbooks mentioned above and the list of references.
THE CONCEPT OF TOXIN-INDUCED PORES
This concept concerns a general process of cell membrane disorganization elicited by a great number of proteins of either prokaryotic origin (bacterial toxins) or from eukaryotic organisms (complement, perforin, animal venoms and toxins, etc.).
This process involves the formation of hydrophilic pores (channels) in the cytoplasmic membrane of target cells provoked by the insertion of these proteins into the membrane, followed by an increase of cell membrane permeability and thereby cell destruction (lysis).
Historically, the first proposal that proteins may create pores in cell membranes concerns the mechanism of erythrocyte lysis by insertion of the C5–C9 components (“membrane complex attack”) of plasma complement described by Mayer (1972) and later on by Bhakdi and Tranum-Jensen (1984).
This process was rapidly extended to an increasing number of cytolytic bacterial protein toxins. Several lines of experimental evidence are consistent with the following modus operandi:
The toxins are released by the bacteria as water-soluble monomeric proteins and then undergo a series of changes upon interacting with target cell membranes. Toxin binding to the membranes facilitates the concentration of the monomers and their transition to form noncovalently associated oligomers. This process leads to an amphipathic state (β-barrel profile) of the oligomers followed by insertion into the membrane and the formation of protein-lined pores of various sizes depending on the toxin involved. In these pores, the hydrophobic sides of toxin molecules are exposed to the lipid fatty acid chains and the hydrophilic sides line up the pore (Freer and Birkbeck 1982; Bhakdi and Tranum-Jensen 1988; Menestrina et al. 1995; Bhakdi et al. 1996; Parker et al. 1994; Bayley 1997; Lesieur et al. 1997; Gouaux 1997).
Visualization of membrane-associated oligomers of pore-forming toxins
Since the pioneering electron-microscopic studies of Dourmashkin and Ross (1966) on erythrocyte damage by complement and streptolysin O, several investigations on PFT have been reported. These authors and many others (see Bhakdi and Tranum-Jensen 1988; Bernheimer and Rudy 1986) observed the formation of ring- and arc-shaped structures by electron microscopy of various cholesterol-binding toxins, particularly streptolysin O, pneumolysin and perfringolysin O. The oligomeric structures on membranes damaged with streptolysin contained about 50 subunits (Alouf 2000). The pores formed by S. aureus α-hemolysin were also observed by electron microscopy (Vandana et al. 1997).
Size of the toxin-induced pores
The pores elicited by the toxins differ widely in size. The smallest pores formed in mammalian cell membranes have been sized to around 1–2 nm effective diameter. This is the case for the S. aureus α-toxin (Bhakdi and Tranum-Jensen 1988; Valeva et al. 1997; van der Goot 2000), E. coli α-hemolysin (Menestrina et al. 1987; Menestrina and Vécsey-Semjén 1999), Aeromonas aerolysin (Buckley 1999; van der Goot 2000), and Vibrio El Tor hemolysin (Zitzer et al. 1997; Shinoda 1999). In contrast, transmembrane pores formed by cholesterol-binding toxins are up to 25–30 nm in diameter (Alouf 2001).
THREE-DIMENSIONAL STRUCTURE OF PORE-FORMING TOXINS
The establishment by X-ray crystallography in the past eight years of the three-dimensional structure of five pore-forming toxins has been reported. It concerned Aeromonas hydrophila proaerolysin (Parker et al. 1994), C. perfringens perfringolysin O (Rossjohn et al. 1997), Staphylococcus aureus α-toxin (Song et al. 1996) and leukocidins Luk F (Olson et al. 1999) and Luk F-PV (Pedelacq et al. 1999). These achievements greatly contributed to a better understanding of the structure activity relationship of these toxins. Interestingly, S. aureus α-toxin and the leukocidins which are distant in sequence were found to be similar in structure (Gouaux 1997).
TYPOLOGY OF PORE-FORMING TOXINS
The progress realized in the cloning and sequencing of PFT structural genes and our present knowledge of their mechanism of action at cellular and molecular levels allows a rational classification of these cytolysins into two categories as initially suggested by Bernheimer and Rudy (1986): “congeneric” and “individualistic” toxins produced by various Gram-positive and Gram-negative bacteria or both. The former category comprises those proteins that share close structural and/or similar physicochemical and functional lytic properties and mechanisms of action while the latter concerns PFT exhibiting an appreciable degree of individuality or uniqueness. These individualistic cytolysins will not be described in this review. A selection of some representative congeneric PFT is listed in Table I.
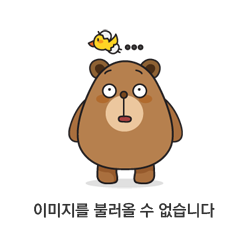
MOLECULAR CHARACTERISTICS
The majority of PFT are simple single-chain proteins secreted by the bacteria (extracellular toxins) but some of them may remain associated at the surface of these microorganisms (e.g., cell-bound form of streptolysin S, Bordetella pertusis adenylate cyclase hemolysin) or remain located in the cytoplasm (intracytoplasmic toxins, e.g., pneumolysin from Streptococcus pneumoniae). The molar mass of PFT ranges from about 2.5 kDa for S. aureus δ-toxin (Alouf et al. 1989; Dufourcq et al. 1999) to some 177 kDa for Bordetella pertusis adenylate cyclase hemolysin (Locht 1999; Ludwig and Goebel 1999). However, many PFT show a broad diversity of molecular topology, complex genomic encoding, structural architecture and functional originality. Some selected families of PFT are described below.
THE FAMILY OF CHOLESTEROL-BINDING CYTOLYSINS
This family is a prototype of simple single-gene-encoded pore-forming toxins. These 50–60-kDa single-chain proteins formerly known as SH-activated toxins, constitute the largest group of structurally related bacterial toxins (for recent reviews see Morgan et al. 1996; Alouf 1999, 2000; Billington 2000; Tweten et al. 2001). The cholesterol-binding cytolysin (CBC) family comprises so far 21 toxins produced by 23 bacterial species of the genera Streptococcus, Bacillus, Brevibacillus, Paenibacillus, Clostridium, Listeria and Arcanobacterium (Table II).
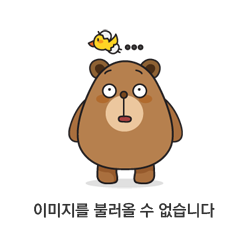
These toxins are secreted in the extracellular medium, except pneumolysin which remains intracytoplasmic (absence of signal peptide) and is only released upon bacterial lysis. Among the CBC-producing species only L. monocytogenes and L. ivanovii are intracellular pathogens which grow and release their toxins in the phagocytic cells of the host. CBC share many features. They are antigenically related, lethal to animals and highly lytic toward eukaryotic cells, including erythrocytes. Their lytic and lethal properties are suppressed by mercapto-blocking agents and reversibly restored by thiols or other reducing agents; these properties are irreversibly suppressed by very low concentrations (nmol/L) of cholesterol and other 3β-hydroxysterols. Membrane cholesterol is thought to be the toxin-binding site at the surface of target eukaryotic cells. Toxin molecules bind as monomers to membrane surface with subsequent oligomerization (c. 50 monomers) into arc- and ring-shaped structures surrounding large pores generated by this process (Morgan et al. 1996; Sekiya et al. 1996; Bayley 1997; Alouf and Palmer 1999; Gilbert et al. 1999; Shatursky et al. 1999; Tweten et al. 2001).
Interestingly, the eukaryotic sea anemone Metridium senile produces a cholesterol-binding cytolysin sharing several biological properties with bacterial CBC, including the formation of arc- and ring-shaped structures on the surface of target cells (Bernheimer et al. 1979). The 13 structural genes of the CBC toxins cloned and sequenced to date (Table II) are all chromosomal and the primary structure of the proteins deduced from the nucleotide sequence of encoding structural genes shows obvious sequence homology, particularly in the carboxy-terminal part and a characteristic consensus common sequence (undecapeptide) containing a unique Cys residue (ECT GLA WEW WR) except for pyolysin and intermidilysin which do not contain this residue which was for a long time considered to be absolutely necessary for biological activity. Indeed, the genetic replacement of the Cys residue in the undecapeptide by certain amino acids demonstrated that this residue is not per se essential for toxin function (Alouf 2001; Tweten et al. 2001). Other residues in the undecapeptide have been mutagenized, particularly the Trp residues (Trp 436 in pneumolysin, Trp 466 and 467 in listeriolysin O and Trp 436, 438 and 439 in perfringolysin O) appeared critical for lytic activity (Alouf 1999; Tweten et al. 2001). Elucidation of the 3-D structure of perfringolysin O provided important information on the structure–activity relationship of this CBC which appeared to comprise four domains (Rossjohn et al. 1997). The Trp-rich region in the undecapeptide within domain 4 is assumed to conformationally adapt to cholesterol.