Introduction
Multicellular organisms exist as meta-organisms comprised of both the macroscopic host and its symbiotic commensal microbiota. With an estimated composition of 100 trillion cells, human symbionts outnumber host cells by at least a factor of 10 and express at least 10-fold more unique genes than their host’s genome (Ley et al., 2006a). These complex communities of microbes that include bacteria, fungi, viruses, and other microbial and eukaryotic species provide a tremendous enzymatic capability and play a fundamental role in controlling many aspects of host physiology.
Over the past few years, the field of immunology has been revolutionized by the growing understanding of the fundamental role of the microbiota in the induction, education, and function of the mammalian immune system. The immune system is composed of a complex network of innate and adaptive components endowed with an extraordinary capacity to adapt and respond to highly diverse challenges. Collectively, this cellular network acts as a formidable regulator of host homeostasis that operates to sustain and restore tissue function in the context of microbial and environmental encounters. The development of defined arms of the immune system—and, more particularly, those associated with adaptive immunity—has coincided with the acquisition of a complex microbiota, supporting the concept that a large fraction of this machinery has evolved as a means to maintain a symbiotic relationships with these highly diverse microbial communities.
In turn, the microbiota promote and calibrate multiple aspects of the immune system. When operating optimally, the immune system-microbiota alliance interweaves the innate and adaptive arms of immunity in a dialog that selects, calibrates, and terminates responses in the most appropriate manner. However, both the acquisition of a complex immune system and its reliance on the microbiota came at a price. Pathologies that increasingly affect humans, such as allergies, autoimmune, and inflammatory disorders, all arise from a failure to control misdirected immune responses against self, microbiota-derived, or environmental antigens. Further, alteration of the composition and function of the microbiota as a result of antibiotic use, diet evolution, and recent elimination of constitutive partners such as helminth worms has transformed our microbial allies into potential liabilities. Although members of the microbiota are often referred to as commensals, symbiosis between the microbiota and its mammalian host encompasses various forms of relationship, including mutualistic, parasitic, or commensal. However, how defined members of the microbiota interact with their host can be highly contextual, with the same microbe developing as mutualist or parasite according to the nutritional, coinfection, or genetic landscape of its host. Over the past decade, exploration of optimal and dysregulated partnerships between the microbiota and its mammalian host has taken center stage in the field of immunology and has led to the rediscovery of a more holistic view of host physiology. Indeed, the notion that microbial partners can promote human health is not a recent concept and was originally proposed by the seminal work of Do¨ derlein (1892) and his understanding of the role of lactobacilli as gatekeepers of the vaginal ecosystem as well as the observation of Metchnikoff associating prolonged life with fermented milk products. Recent sequencing efforts of the human metagenome have changed our understanding of the microbiome and how variations in these populations can contribute to disease states. In this Review, we will discuss some of the major concepts that have emerged from the recent dialog between immunologists, geneticists, microbiologists, and clinicians that highlight the complex role of the microbiota on the immune system in health and diseases.
Microbiota-Immune System Interaction during Development
Under normal conditions, the fetal gastrointestinal tract is believed to be sterile, with the first exposure of the immune system to commensals occurring during the passage through the birth canal. These early interactions are considered to set the tone of the mucosal and systemic immune system for the long term. The mechanism by which neonate tissues adapt to the formidable challenge of microbial colonization remains incompletely understood, but factors contained in maternal milk are believed to define some of these early responses to commensals. Indeed, colostrum and breast milk contain live microbes, metabolites, IgA, and immune cells as well as cytokines. These factors synergize to shape the breast-fed infant microbiota and the response of the host to these microbes.
For instance, maternal IgA restricts immune activation and microbial attachment by binding nutritional and microbial antigens, and the presence of metabolites, including oligosaccharides in mother’s milk, promotes the expansion of defined constituents of the microbiota such as Bifidobacterium (Marcobal et al., 2010; Marcobal and Sonnenburg, 2012). Bacterial translocation from the mouse gut is increased during pregnancy and lactation, and bacterially loaded dendritic cells in the milk have been proposed to contribute to neonatal immune imprinting (Perez et al., 2007). The capacity to accept the microbiota can also be explained by the relative immaturity of the neonate immune system at birth and the tolerogenic environment that defines early mammalian life. Indeed, the developing immune system is characterized by blunted inflammatory cytokine production and skewed T and B cell development in favor of regulatory responses (PrabhuDas et al., 2011; Siegrist, 2001).
Although a consequence of this blunted immune response is high susceptibility to infections, this regulatory environment ensures that the establishment of the microbiota occurs without overt inflammation. Recent reports reveal that a defined population of erythroid cells enriched in neonates contributes to the maintenance of this immunoregulatory environment and limits mucosal inflammation following colonization with the microbiota (Elahi et al., 2013). Early exposure of the host to commensals can also repress cells involved in the induction of inflammatory responses such as invariant natural killer T (iNKT) cells, an effect that has long-term consequences for the host capacity to develop inflammatory diseases (Olszak et al., 2012). A recent report proposed that this control can be mediated by the direct interaction, early in life, of unique inhibitory commensal-derived sphingolipids with iNKT cells (An et al., 2014).
One of the primary modes of dialog between the host and the microbiota is mediated by the recognition of conserved microbial-associated molecular patterns (MAMPs). The neonate innate immune system integrates these signals in a unique way to promote healthy microbial colonization. For instance, although neonate innate cells express Toll-like receptor (TLR) ligands, their response to microbial ligands is distinct from that of adult cells, with notable impairment in the production of inflammatory mediators such as oxygen radicals and heightened production of regulatory cytokines such as IL-10 (Kollmann et al., 2012). Part of this phenomenon results from the action of the microbiota itself. Indeed, early responses to microbial ligands such as LPS, the endotoxin found in the outer membrane of Gram-negative bacterial walls, condition gut epithelial cells to become hypo-responsive to subsequent TLR stimulation (Chassin et al., 2010; Lotz et al., 2006).
How the innate immune system integrates microbial-derived signals remains unclear, but recent findings support the idea that expression of epigenomemodifying enzymes by epithelial cells may be required for the coordination of commensal dependent intestinal homeostasis (Alenghat et al., 2013). Commensals also contribute to the postnatal development of the immune system that, in turn, contributes to their containment. Studies performed in animals raised in the absence of live microbes referred to as germ-free (GF), revealed that the microbiota plays a critical role in secondary lymphoid structure development. This is particularly evident in the gastrointestinal tract, where germ-free mice are characterized by smaller Peyer’s patch size and a reduced number of CD4+ T cells and IgA-producing plasma cells (Bauer et al., 1963; Hamada et al., 2002; Macpherson et al., 2001; Mazmanian et al., 2005; Smith et al., 2007; Talham et al., 1999). In the intestine, tertiary lymphoid structures such as isolated lymphoid follicle or crytopatches are induced after birth as a result of commensal exposure (Bouskra et al., 2008; Ohnmacht et al., 2011). As further discussed below, commensals can also contribute to the fortification of the intestinal barrier by various mechanisms, including the promotion of epithelial cell maturation and angiogenesis (Hooper et al., 2001; Stappenbeck et al., 2002). When operating properly, the highly regulatory tone of the neonate immune system and the action of commensals in the development and training of this system lead to the establishment of a durable and homeostatic host/commensal relationship. These primary encounters between the host immune system and the microbiota have profound and long-term implications for human health. Indeed, epidemiological observations revealed that alteration of the microbiota in mothers or in neonates may predispose to diseases associated with dysregulated barrier responses, such as asthma (Ege et al., 2011).
Containing the Microbiota
An important point to consider when exploring the role of the microbiota on the immune system is that pathogenicity is, in most cases, a contextual state. Indeed, the capacity of a given microbe, including those composing the microbiota, to trigger or promote disease is highly dependent on the state of activation of the host, the host’s genetic predisposition, and the localization of the particular microbe. As such, the mechanisms utilized by the immune system to maintain its relationship with the microbiota are highly analogous to the ones that are used to constrain organisms with pathogenic potential. An enormous fraction of the immune system’s constitutive function is aimed at controlling our relationship with the microbiota. As such, the highest number of immune cells in the body are resident at sites colonized by commensals such as the skin or the GI tract.
In turn, to protect their ecological niche, a dominant action of the healthy microbiota on the immune system is aimed at reinforcing barrier immunity and therefore their own containment. A central strategy utilized by the host to maintain its homeostatic relationship with the microbiota is to minimize contact between microorganisms and the epithelial cell surface, thereby limiting tissue inflammation and microbial translocation.
In the gastrointestinal tract, home to the largest density of commensals, this segregation is accomplished by the combined action of epithelial cells, mucus, IgA, antimicrobial peptides, and immune cells. Collectively, these structural and immunological components have been referred to as the ‘‘mucosal firewall’’ (Figure 1) (Macpherson et al., 2009).
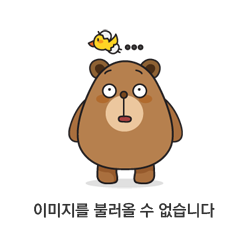
Figure 1. The Mucosal Firewall (1) The mucus represents the primary barrier limiting contact between the microbiota and host tissue preventing microbial translocation. (2) Epithelial cells produce antimicrobial peptides that also play a significant role in limiting exposure to the commensal microbiota. (3) Translocating commensals are rapidly eliminated by tissue-resident macrophages. (4) Commensals or commensal antigens can also be captured by CD103+ CD11b+ DCs that traffic to the mLN from the lamina propria but do not penetrate further. Presentation of commensal antigens by these DCs leads to the differentiation of commensal-specific regulatory cells (Treg), Th17 cells, and IgA-producing B cells. Commensal-specific lymphocytes traffic to the lamina propria and Peyer’s patches. In the Peyer’s patches, Treg can further promote class switching and IgA generation against commensals. The combination of the epithelial barrier, mucus layer, IgA, and DCs and T cells comprises the ‘‘mucosal firewall,’’ which limits the passage and exposure of commensals to the gut-associated lymphoid tissue, preventing untoward activation and pathology
The mucus represents the primary shield limiting contact between the microbiota and host tissue and preventing microbial translocation (McGuckin et al., 2011). In addition to the production of mucus by goblet cells, all intestinal epithelial cell lineages can produce antimicrobial peptides that play a significant role in limiting exposure to the commensal microbiota (Hooper and Macpherson, 2010). These proteins can exert antimicrobial functions resulting from enzymatic attack of the bacterial cell wall or by disrupting the bacterial inner membrane (Hooper and Macpherson, 2010). Some of these molecules, such as a-defensins, are constitutively expressed by epithelial cells, whereas in other cases, engagement of pattern recognition receptors (PRRs) by commensally derived products is required (Hooper and Macpherson, 2010). One of the best-characterized mucosal antimicrobial peptides is RegIIIg, which is expressed soon after birth or following colonization of germ-free mice (Cash et al., 2006). Production of this lectin is tightly controlled by the flora in a MyD88-dependent manner and has a direct microbicidal effect on Gram-positive bacteria (Brandl et al., 2007; Cash et al., 2006; Ismail et al., 2011; Mukherjee et al., 2009).
Accumulation of antimicrobial peptides such as RegIIIg in the mucus contributes to the maintenance of the segregation between the microbiota and the host intestine, creating a physical separation referred to as the ‘‘demilitarized zone’’ (Vaishnava et al., 2011). Compartmentalization of intestinal bacteria also depends on secreted immunoglobulin A (IgA). IgA specific for commensals is produced with the help of intestinal dendritic cells that sample commensals associated with the epithelium and interact with B and T cells in the Peyer’s patches to produce IgA specific for commensal-derived antigens (Macpherson and Uhr, 2004). Further, commensals that translocate across the intestinal epithelial cell barrier can be rapidly engulfed and eliminated by macrophages that reside in the lamina propria or carried alive by dendritic cells (DC) (Kelsall, 2008; Macpherson and Uhr, 2004). The bacteria loaded DC traffic to the mesenteric lymph node via the intestinal lymphatics but do not penetrate further, allowing the induction of a mucosal-compartmentalized IgA response (Macpherson and Uhr, 2004). IgA+ B cells migrate to the intestinal lamina propria and secrete IgA that are subsequently transcytosed across epithelial cells. These transcytosed IgAs control host commensal interaction by both impacting commensal gene expression (Peterson et al., 2007) and preventing adhesion of commensal bacteria to the epithelial surfaces (Figure 1) (Boullier et al., 2009; Fernandez et al., 2003; Hornquist and Lycke, 1995; Martinoli et al., 2007; Wade and Wade, 2008; Wei et al., 2011). Mucosal IgA responses lack classical memory characteristics and are able to respond to flux in commensal microbiota composition. Indeed, established IgA-producing clones are outcompeted by novel antibacterial responses, allowing the mucosal immune system to respond to a constantly changing microbiota (Hapfelmeier et al., 2010).
Most activated or memory T cells reside in tissues that are constitutively colonized by commensals such as the skin and the GI tract. Notably, at steady state, most IL-17 (Th17) and IFNg-producing (Th1) T cells are found in the GI tract and develop from signals that are derived from the microbiota (Gaboriau-Routhiau et al., 2009; Ivanov et al., 2008; Macpherson and Harris, 2004). The current view is that constitutive sensing of commensals plays an important homeostatic role, whereas active responses against the flora are believed to be associated with pathogenesis. However, this distinction is clearly not absolute and needs to be revisited in light of the observation that healthy human serum normally contains antibodies and T cells specific to commensals (Ergin et al., 2011; Macpherson et al., 1996), suggesting that a certain degree of commensal recognition is a common occurrence and, in most circumstances, is not associated with pathogenic responses.
Although tissue-derived cues can dictate the induction and maintenance of Th17 cells irrespective of antigen specificity, we could speculate that, in a similar manner to that proposed for Treg cells, antigenic specificities of tissue-resident effector T cells are highly enriched for commensal antigens.
Notably, Th17 cells produce cytokines such as IL-17 and, more particularly, IL-22, which contribute to the homeostatic dialog with commensals via the capacity of these cytokines to act on epithelial cell function. Failure to maintain the Th17 lineage in the gut, as observed during HIV or SIV infection, is associated with microbial translocation that contributes to dissemination of the virus (Klatt et al., 2013). The action of IL-22 on the mucosal immune system is highly pleiotropic and promotes the production of antimicrobial peptides, enhances of epithelial regeneration, increases mucus production, and regulates wound repair (Wolk and Sabat, 2006; Zenewicz and Flavell, 2011). This cytokine can also be produced by other cell lineages and, more particularly, by a population of gut-resident innate Figure 2.
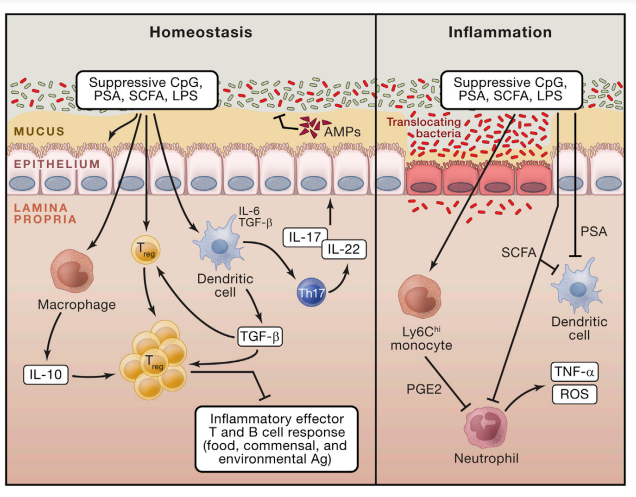
Figure 2. Promotion of Immune Regulation by the Microbiota during Steady State and Inflammation (Left) Commensals promote the induction of regulatory T cells via direct sensing of microbial products or metabolites by T cells or dendritic cells. Further commensals promote the induction of Th17 cells that can regulate the function and homeostasis of epithelial cells. In the context of inflammation, similar mechanisms may account for the regulatory role of the microbiota. (Right) Commensal-derived metabolites can also have a local and systemic effect on inflammatory cells. For example, SCFA can inhibit neutrophil activation. Upon entrance in the tissue, inflammatory monocytes can also respond to microbial-derived ligands by producing mediators such as PGE2 that limit neutrophil activation and tissue damage.
Promotion of Immune Regulation by the Microbiota during Steady State and Inflammation (Left) Commensals promote the induction of regulatory T cells via direct sensing of microbial products or metabolites by T cells or dendritic cells. Further commensals promote the induction of Th17 cells that can regulate the function and homeostasis of epithelial cells. In the context of inflammation, similar mechanisms may account for the regulatory role of the microbiota. (Right) Commensal-derived metabolites can also have a local and systemic effect on inflammatory cells. For example, SCFA can inhibit neutrophil activation. Upon entrance in the tissue, inflammatory monocytes can also respond to microbial-derived ligands by producing mediators such as PGE2 that limit neutrophil activation and tissue damage. cells referred to as group 3 innate lymphoid cells (ILCs). Although some reports propose that the development of these cells is independent of signals derived from the microbiota, their phenotype and functional capacity can evolve to accommodate physiologic alterations in the intestinal environment following microbial colonization at birth (Satoh-Takayama et al., 2008). Production of IL-22 by ILC promotes the containment of specific members of the microbiota community and, more particularly, microbes that reside in mucosal lymphoid structures, such as bacteria of the Alcaligenes genus (Qiu et al., 2013; Sonnenberg et al., 2012). Thus, in addition to broad and nonspecific modes of commensal containment, discrete pathways may have evolved to promote the selective containment of communities of microbes residing in unique ecological niches.
'Role of the Microbiota in Immunity and' 카테고리의 다른 글
Role of the Microbiota in Immunity and Inflammation2 (0) | 2024.12.24 |
---|